Purpose
Iridium-192 (192Ir) high-dose-rate (HDR) brachytherapy (BT, interventional radiotherapy) is a well-established technique for the treatment of large number of tumors [1]. Compared with other types of radiotherapy, BT is particularly advantageous in skin tumors due to the use of specific skin applicators that can be personalized for each patient. In particular, BT is an effective treatment method for non-melanoma skin cancer (NMSC), with catheters being implanted inside the tumor or on the skin. Treatment planning is usually straightforward but at present, dose calculation is performed with an algorithm based on the TG-43 formalism, which considers a homogenous all-water environment [2-4]. In 2012, the Task Group 186 report on model-based dose calculation methods has been published, presenting the current status and recommendations for clinical implementation [5]. Recently, a joint AAPM, ESTRO, ABG, and ABS report on commissioning of model-based dose calculation algorithms in BT has been published, highlighting the importance of implementing such tools in clinical practice [6].
Several authors have investigated how the TG-186 model would influence dose calculation [7, 8] in order to understand clinical impact. As reported by Boman et al. [9], TG-43 dosimetry formalism cannot model surface mold treatments in the absence of full scatter conditions within 5% for loading areas larger than approximately 5 × 5 cm2. The TG-43 model results in an overestimation of delivered dose, which increases with treatment area. This confirms the need for model-based dose calculation algorithms, as discussed in TG-186.
The purpose of this retrospective study was to compare treatment plans for NMSC BT calculated with the TG-43 protocol and with the advanced collapsed cone engine (ACE, Elekta Stockholm, Sweden) TG-186 model-based dose calculation algorithm (MBDCA). The study focused on assessing dosimetric differences between the two approaches for NMSC patients undergoing interstitial brachytherapy. Furthermore, the impact of the introduction of a bolus was investigated in order to mimic the presence of water outside the body, as it was considered for calculation with the TG-43 algorithm.
Material and methods
For our retrospective dosimetric analysis, we selected ten treatment plans designed for patients with NMSC affecting the eyelid. Patients received treatment with plastic interstitial catheters (median, 2; range, 2-4 catheters). The chosen treatment modality was high-dose-rate (HDR) 192Ir BT. Each patient underwent a CT scan, conducted with a slice thickness of 0.625 mm using a Discovery CT590RT CT scanner (GE Medical Systems).
Contouring of clinical target volume (CTV) and organs at risk (OARs) was carried out manually by an experienced radiation oncologist using Oncentra Brachy TPS v. 4.6 (Elekta). CTV volumes ranged from 0.39 to 2.92 cc, with a mean of 1.2 ±0.7 cc, and dose non- homogeneity ratio (DNR) was calculated as the ratio between V150 and V100. Catheters were also manually reconstructed, and two treatment plans were created. The first plan utilized the TG-43 algorithm, while the second plan was calculated using the TG-186 algorithm with standard accuracy (advanced collapsed cone, ACE; Elekta). Importantly, dwell times, positions, and calculation grid size remained consistent between these two plans. The average number of dwell positions for these plans was equal to 83 ±40.
In the model-based approach of TG-186 algorithm, specific material types and Hounsfield unit values must be assigned for each region of interest (ROI). In this study, CTV was defined as soft tissue material, while OARs (specifically, the homolateral lens, optic nerve, and eye) were categorized as “eye lens”. To account for external air surrounding the patient, a 3 cm air structure was created around the body, assigned “air” material, with a uniform mass density of 0.001 g/ml (Figure 1A, air represented in cyan).
Fig. 1
A) The CT slice of a NMSC patient with CTV and OARs contoured. A 3 cm layer of air, shown in cyan, was added externally with a mass density of 0.001 g/ml, while 0 cm bolus was considered. B-J) The same CT slice, with an addition of bolus with varying thicknesses from 0.25 to 5 cm. This electronic bolus, shown in orange, was considered as water with a mass density of 1 g/ml
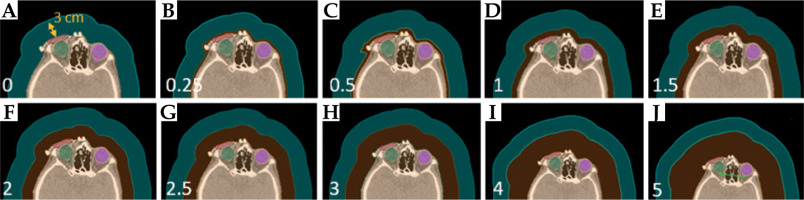
To assess differences in doses calculated by the TG-43 and TG-186 algorithms, we examined clinically relevant dosimetric parameters and conducted a t-Student test. For CTV, V150%, V100%, V95%, and V90% were considered, representing volumes receiving 150%, 100%, 95%, and 90% of the prescription dose, respectively. Additionally, the dose delivered to a 2 cc volume (D2cc) for the homolateral eye was calculated, and for the homolateral lens and homolateral optic nerve, the dose to a 0.01 cc volume (D0.01cc) was recorded.
Furthermore, computing time for the dose calculation time for the TG-186 algorithm across all ten patients was evaluated and compared with relative number of active dwell positions used in the treatment plans.
As a second phase of our study, we utilized a CT scan of one of ten patients to investigate how the introduction of a bolus would impact dose distribution. Specifically, we generated ten plans by adding an electronic water bolus with varying thicknesses, ranging from 0 to 5 cm (as depicted in Figure 1B-J, with boluses of different thicknesses shown in orange). Electronic bolus was incorporated around the patient’s head for comprehending dose distribution relative to thickness. Although it may deviate from the bolus employed in clinical practice, this methodology is used for ascertaining the optimal thickness for this specific treatment.
For TG-186 calculations, water as the material for this bolus was assigned, with a uniform mass density of 1 g/ml.
Results
Table 1 presents a visual representation of the dosimetric and volumetric findings derived from our comparison of the TG-43 and TG-186 algorithms. Our investigation showed several crucial observations. Notably, there was evident and consistent decrease in all volumetric parameters concerning clinical target volume (CTV) when employing the TG-186 algorithm. This pattern remained consistent across different parameters, with the most notable and statistically significant impact observed in V95CTV. The mean values of dose non-homogeneity ratio resulted in 0.59 ±0.1 for TG-43, and 0.55 ±0.1 for TG-186.
Table 1
Summary of mean, median, and minimum values of dosimetric and volumetric parameters for CTV and OARs calculated with TG-43 and model-based TG-186 algorithms
The impact of this decline in CTV coverage is of particular importance since our analysis revealed that CTV coverage below the critical 95% threshold was observed in 6 out of the 10 patients when the TG-186 algorithm was utilized. This outcome underscores the importance of algorithm selection in radiation therapy planning, especially when maintaining adequate CTV coverage is of paramount concern.
In contrast, our study unveiled a more reassuring outcome in the range of doses administered to OARs. Here, both the TG-43 and TG-186 algorithms demonstrated comparable performance, indicating that the choice of algorithm had a lesser impact on OARs doses.
Calculation times, which were notably substantial, were another aspect of the study. While the TG-43 dose calculation provided immediate results, the computation time for dose calculation using the TG-186 was close to 176.5 minutes. Importantly, we observed a clear and expected linear correlation between the calculation time and the number of dwell times employed in the treatment planning process.
Table 2 provides a comprehensive overview of our calculation time findings, and highlights a crucial practical consideration. It becomes evident that, for cases involving more than 50 dwell positions, calculation times associated with the TG-186 algorithm reach durations that are simply incompatible with routine clinical practice. These extended calculation times can lead to significant logistical challenges, and could impede timely delivery of treatments.
Table 2
Computing time for TG-186 algorithm as a function of the number of active dwell positions for 10 patients considered in the study
Calculation time (min) | Dwell positions (n) |
---|---|
60 | 29 |
90 | 50 |
120 | 63 |
160 | 53 |
180 | 75 |
190 | 86 |
200 | 94 |
210 | 105 |
290 | 102 |
330 | 173 |
These findings underscore the importance of optimizing treatment planning processes and considering alternative approaches, especially when dealing with complex cases, which involve a high number of dwell times. Balancing accuracy with efficiency in dose calculations is essential to ensure that patients receive timely and effective care.
The second phase of our research assessed the impact of introducing varying thicknesses of bolus material. In order to accomplish this phase, we retrospectively employed a patient’s CT data to calculate doses using both the TG-43 and TG-186 algorithms for different bolus thicknesses. Our primary focus for comparing these algorithms was on V95CTV. As expected, the TG-43 algorithm exhibited consistent results across different bolus thicknesses, as indicated by the circular data points in Figure 2. Conversely, when employing the TG-186 algorithm, we observed a noteworthy trend: the V95CTV increased as the bolus thickness varied, denoted by the triangular data points. Specifically, when utilizing a water bolus with a thickness of 2 cm, the differences in V95CTV between the TG-43 and TG-186 algorithms were reduced to less than 0.5%.
Discussion
Modern BT for skin cancer relies on precise dose shaping; therefore, it requires an extremely accurate dose calculation [10]. However, the most clinically available treatment planning systems used for BT dose calculation are based on the AAPM TG-43 formalism that does not adequately account for the backscatter defect [11, 12]. Granero et al. investigated the impact of this approximation on skin BT considering the addition of equivalent tissue material (bolus) both in the case of contact BT and interstitial BT over a custom mold to compensate for the backscatter defect of 192I using Monte Carlo calculations [13]. They concluded that personalized approach is essential, as there is no universally applicable solution for each patient and treatment. Nevertheless, they underscored that the TG-186 calculation holds paramount significance, and must be unequivocally addressed. Other authors confirmed the emerging interest within clinical community towards the use of TG-186 model-based dose calculations as complementary to the use of TG-43, both for verification purpose and quality improvement [14].
In the present study, the choice to investigate patients treated for eyelid NMSC with interstitial BT was accurately taken after considering several clinical factors. In fact, BT for NMSC may be delivered either through contact or interstitial approach, according to anatomical location, size, and histopathological risk factors. Particularly, in the case of face NMSC, high-risk zones can be identified, including eyelids, nose vestibule, and lips, which usually require an interstitial approach [15]; on the contrary, for non-high-risk zones, contact BT can be used. However, for the current study, we considered that contact BT would present additional confounding factors in calculations because molds can be home-made [16], customized from commercially available flaps [1], or even 3D-printed [17, 18]. Regarding specific anatomical subsite of high-risk NMSC, we decided that the eyelid was the ideal choice both from practical point of view and for clinical relevance of the findings.
Concerning practical advantages, the time required to perform calculations using the TG-186 formalism is superior to that of the TG-43, and obviously increase with larger volume [19]. In the case of eyelid patients, clinical target volumes (CTVs) are typically smaller compared with other high-risk zones, which allow to perform calculations without affecting clinical practice. Another point to consider is the fact that even though CTVs are quite small, plastic tubes implanted within CTV could be placed on a monolayer, and values of DNR resulted to be comparable with larger CTVs requiring multiple planes of implant [20, 21]. When considering the clinical relevance of our findings, the main point to highlight is that eyelids are a challenging anatomical location for different reasons. The volume to be treated is established according to a curved spatial arrangement, and TG-186 has proven to be more reliable compared with TG-43, especially in complex volumes [22]. Another aspect of paramount significance is the strict closeness of CTV to the eye that is a sensitive organ at risk (OAR) to spare. Such anatomical condition where CTV is practically attached to OAR is absolutely unique for NMSC eyelid tumors, and even small variations in CTV coverage are clinically relevant. In particular, the doses used in our series are in line with those reported in other papers. In the study, we delivered 3.5 Gy twice a day in 14 fractions, with a total dose of 49 Gy [23]. In addition, there is evidence from previous clinical experiences that such interstitial treatment is well-tolerated, with a good level of compliance and no major complications reported in largest series [24]. Even though there are no guidelines on the constraints in the case of the eye, the ocular structures to consider as OARs include lacrimal gland, cornea, eye lens, retina, and optic nerve [25].
In this study, we found that the TG-186 algorithm resulted in less coverage for CTV, shown in every dosimetric parameter considered for CTV, with a statistically significant decrease in V95CTV. The number of patients considered was not substantial, but it is very important to evaluate the impact of calculation approximations on such small areas, especially because they are close to air. For this kind of treatment, we found that the introduction of a bolus would decrease the difference in calculation between the TG-43 and TG-186 algorithms. With such a bolus, the TG-43 resulted in dose calculations accurate to less than 0.5%. Therefore, this study shows that for NMSC treatments, the introduction of a bolus may be beneficial when MBDCA are not available, or when it is not possible to cope with their longer calculation times.
In this comprehensive study, we uncovered significant insights into the TG-186 algorithm’s performance in the context of CTV coverage. Across all dosimetric parameters considered for clinical target volume (CTV), we consistently observed a reduction in coverage when employing the TG-186 algorithm, with a particularly pronounced and statistically significant decrease in V95. In contrast, our investigation revealed a more favorable results concerning doses delivered to organs at risk (OARs). In this aspect, both the TG-43 and TG-186 algorithms exhibited similar results, suggesting that the choice of algorithm had a diminished influence on OARs doses. The equivalence in OARs dosing outcomes implies that the emphasis on algorithm selection may need to be predominantly placed on CTV coverage, where the impact of algorithm choice is more considerable.
While the patient cohort in this study was not extensive, it is crucial to emphasize the importance of assessing the impact of calculation approximations on such small areas, especially when they are situated near air interfaces.
Interestingly, our investigation revealed a potential remedy for discrepancies between the TG-43 and TG-186 algorithms, particularly if a model-based dose calculation algorithm (MBDCA) is not readily available at treatment centers. We found that the use of a 2 cm bolus may mitigate these calculation differences. By introducing such a bolus, our findings indicate that the TG-43 algorithm can yield dose calculations with an accuracy level falling within the margin of less than 0.5%.
Therefore, this study underscores the relevance of considering bolus implementation, especially in the context of NMSC treatments when MBDCA tools are not accessible, or when longer calculation times associated with these advanced algorithms pose practical challenges.
Nevertheless, it is crucial to take into account that the current TG-43 approach has been the foundation of clinical outcomes for decades. Consequently, any modification to clinical practice should be approached with careful evaluation. Prospective trials are desirable to confirm the results obtained in our study.
Conclusions
Brachytherapy stands as a highly effective approach in the treatment of non-melanoma skin cancer. Despite its proven efficacy, the current state of treatment planning lacks clinically accepted calculation algorithm capable of adequately addressing tissue heterogeneities. In this study, we investigated the dosimetric differences between the widely used TG-43 algorithm and more advanced TG-186, focusing on patients with NMSC affecting the eyelid. Our research not only illuminates these differences, but also presents a practical solution for cases where TG-186 is not readily available: the incorporation of a bolus.