Arterial pressure is a key haemodynamic variable that is often continuously monitored in critically ill patients. In shock management, frequent measurement of blood pressure is mandatory [1], and the Surviving Sepsis Campaign recommends a mean arterial pressure (MAP) target of 65 mmHg as a starting point [2]. Persistent hypotension is associated with worse outcomes in septic shock [3].
The advantages of invasive arterial pressure (IAP) monitoring include precision and instantaneous detection of pressure changes, as well as monitoring and treating blood pressure more closely, but it is invasive and has the risk of complications due to arterial cannulation, such as bleeding, haematoma, pseudoaneurysm, infection, nerve damage, and distal limb ischaemia [4, 5]. Noninvasive intermittent arm blood pressure (NIBP) by oscillometric mode is the first-line monitoring technique during immediate admission to the ICU or throughout the ICU stay. During the widespread use of intermittent NIBP, its fundamental operating principles, mainly with regard to the physical principles of MAP, systolic arterial pressure (SAP), and diastolic arterial pressure (DAP), are proprietary, and this may partially explain why the reliability of intermittent NIBP is sometimes questioned, encouraging invasive measurements [6]. However, a large cohort study with more than 60,000 patients found no association between arterial catheter placement and improved prognosis in critically ill patients, even in those who received vasopressors [7].
NIBP is mostly studied in the perioperative setting, but characteristics of the management of critically ill patients, such as infusion of vasopressors and inotropes, oedema, hypoperfusion, shock, and a high incidence of arrhythmia, indicate that a specific assessment of this population is necessary [6, 8, 9]. Therefore, the aim of this study was to assess the accuracy and the precision of NIBP compared with that of invasive arterial monitoring in critically ill patients.
METHODS
This systematic review and meta-analysis was conducted following the recommendations of the Preferred Reporting Items for Systematic Reviews and Meta-analyses (PRISMA) statement [10, 11]. The systematic review protocol was registered with the International Prospective Register of Systematic Reviews (PROSPERO; registration number CRD42018115625).
Eligibility criteria
We included observational studies addressing the research question. The search and subsequent bibliographic review were restricted to studies in adult humans and to published papers (not correspondence or case reports) in English. The popu-lation of interest was critically ill patients aged 18 years or older. Critically ill patients were defined as those treated in a high acuity, critical care, or ICU of any type (e.g. burn, cardiac, surgical, medical, trauma, or mixed), and included studies with patients who were normotensive or using vasoactive drugs. We included studies that evaluated both NIBP and IAP measurements by an arterial catheter simultaneously, in critically ill patients, at any site. NIBP was defined by intermittent oscillometric mode. IAP measurement was defined by the measurement performed by an invasive arterial catheter in femoral, brachial, radial, or axillary sites. The studies had to report their results using bias and precision statistics (mean difference and SD of agreement, respectively). We included only studies using comparisons with simultaneous measurements of arterial pressure by NIBP (intervention) and invasive arterial pressure measurement (control).
Data sources and search strategy
A MEDLINE (via PubMed), CINAHL, and EMBASE search was conducted with the search headings “intensive care unit” and “blood pressure” from 1980 to 2022. Reference lists of relevant articles were reviewed for additional studies not identified by electronic searches.
Study selection and data extraction
Two reviewers (ATV and KAG) independently selected titles and abstracts in duplicate for full-text analysis. The same duplicate, independent review process was followed by reviewing the full text of all potentially eligible articles. The reference data of the retrieved publications were manually searched for potentially eligible studies. Disagreements were resolved by a third reviewer (PRS). Two reviewers (ATV and KAG) independently performed data extraction using a predefined form. Data were extracted on the year of study, the number of patients in each study, the clinical characteristics of the population studied, the type and location of measurement of NIBP, the measurement of the invasive arterial site, the use and dose of vasopressors, the mean, standard deviation and 95% limits of agreement of MAP and their respective standard deviations, both in NIBP and in invasive arterial measurement (IAP – NIBP).
Outcomes
The primary outcome was to define the bias of the MAP measured by NIBP over the IAP measurement. Because the description of bias was not uniform among the studies (some articles described it as noninvasive minus invasive measurements, whereas others described it as invasive minus noninvasive measurements), we standardized bias in the current meta-analysis to mean NIBP minus IAP measurement and corrected the source data as needed for reporting in this form. We defined the accuracy and precision of MAP measurement as acceptable if the bias was not greater than 5 mmHg and the precision was not greater than 8 mmHg, as previously described [8]. We also described the precision (sensitivity, specificity, positive and negative likelihood ratio) of the NIBP measurements in detecting hypotension (defined as MAP < 65 mmHg) when studies reported this result. We performed a secondary analysis estimating the bias of the SAP and DAP measured by NIBP over the IAP measurement when studies reported these data.
Evaluation of study quality
Each data extractor independently assessed the risk of bias of their assigned studies, and a third author (PHS) confirmed the final bias assessment. The Quality Assessment of Diagnostic Accuracy Studies (QUADAS-2) tool [12] was used to assess study quality.
Statistical analysis
The principal summary measures of the current meta-analysis were i. accuracy of measurement (mean difference, defined as noninvasive – invasive measurement) and ii. precision of measurement (defined as standard deviation [SD] of accuracy). For the synthesis of pooled estimates of bias and SD, we used random-effects models. We tested the heterogeneity of biases and SDs across studies using the Cochran Q test and the I2 statistic [13, 14]. Forest plots are presented with individual and random-effects pooled estimates of bias and 95% limit of agreement, to visualize the data. The results are presented in forest plots with 95% confidence intervals (95% CIs). All analyses were performed with the R statistical software version 3.4.4 (R Development Core Team, 2008) with the Meta and Mada package, version 4.8-1.
RESULTS
After screening 2208 titles and abstracts we found 7 studies eligible for this review (Figure 1). Seven studies reported mean bias in the measurements for MAP [15–21] and 6 reported mean bias for SAP and DAP [15–17, 19–21]. The number of patients allocated in these studies ranged from 55 [15] to 736 [21].
All studies assigned patients to vasoactive drugs but were not exclusively centred on this population. The dose of norepinephrine in the studies, when reported, ranged between 0.14 mg kg-1 min-1 [21] and 0.7 mg kg-1 min-1 [17]. The use of other vasoactive drugs was not reported. One study evaluated patients using intravenous nicardipine [20]. The main site for arterial catheter placement was the radial artery [15–21], and 4 studies included patients with arterial catheters at the femoral site [16–19]. One study also evaluated the auscultatory mode [15]. The mean clinical characteristics of the included studies are reported in Table 1.
TABLE 1
Clinical characteristics of included studies
One study evaluated critically ill patients with BMI > 25 kg m–2 [15], 2 studies evaluated clinical-surgical ICU patients [16, 17], one study evaluated maternal ICU patients [20], and 3 studies evaluated only clinical patients [15, 18, 19]. Four studies reported results in patients with sepsis or septic shock [16–19], one study in patients with arrythmia [18], and 5 studies in patients with acute circulatory failure [16–19, 21]. One study evaluated patients with cardiogenic shock [18], and one study evaluated patients with severe peripartum hypertensive disorders [20]. The correlation coefficient between IAP and NIBP varied between studies, and 2 studies reported these data. For MAP, the coefficient varied between 0.81 [19] and 0.85 [16].
A total of 1593 patients were included in the meta-analysis of MAP measurements. MAP measured by the noninvasive method had a mean value of –1.5 mmHg compared to IAP (95% CI: –3.34 to 0.35; I2 = 96% for the random effects model, P < 0.01) (Figure 2). There was, however, a wide amplitude in the 95% limits of agreement when comparing NIBP and IAP. The limits of agreement were wide for MAP varying between –14.6 mmHg [17] and +40.3 mmHg [15] (Table 2).
FIGURE 2
Mean estimate of difference between methods (noninvasive arterial pressure measurement - invasive arterial pressure measurement)
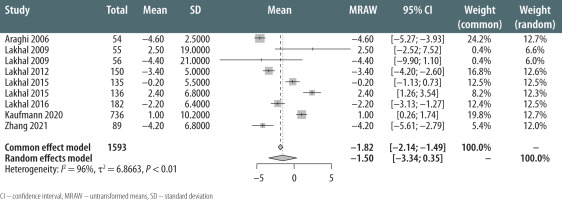
TABLE 2
Accuracy, precision, and limits of agreement of NIBP MAP measurements
Hypotension was defined as MAP < 65 mmHg in 2 studies. In one of them [18], the area under the receiver operating characteristic curve (AUROC) for NIBP-discriminating patients with hypotension was 0.90 (0.71–1.00). With a cut-off at 65 mmHg, the positive likelihood ratio (LR) was 7.7 (5.4–11.0) and the negative LR was 0.31 (0.22–0.44). In another study [16], the 65 mmHg cut-off for noninvasive MAP in the arm had an AUROC of 0.98 (95% CI: 0.92–1.00), with a positive LR of 20 (95% CI: 18–22) and a negative LR of 0.05 (95% CI: 0.01–0.40). Lakhal et al. [18], found a predictive ability of NIBP to detect hypotension (invasive MAP < 65 mmHg) with an AUROC of 0.90 (95% CI: 0.71–1.00) and a positive and negative LR of 7.7 (95% CI: 5.4–11.0) and 0.31 (95% CI: 0.22–0.44), respectively. In another study, evaluating an oscillometric brachial cuff [17], the system had an AUROC of 0.92 (95% CI: 0.90–0.94), sensitivity of 83%, specificity of 90%, and positive and negative LRs of 7.6 and 0.19, respectively. In secondary analysis, the pooled mean bias for SAP was –1.23 mmHg (95% CI: –7.63 to 5.17, I2 = 100% for random effects model, P < 0.01) and the pooled mean bias for DAP was –3.61 mmHg (95% CI: –5.04 to –2.19, I2 = 95% for random effects model, P < 0.01). The QUADAS-2 tool, addressing the quality of the included studies, is presented in Table 3. In general, most studies had a low risk of bias.
TABLE 3
QUADAS 2 of included studies
DISCUSSION
This meta-analysis evaluated the accuracy and precision of oscillometric NIBP monitoring systems compared to IAP measurements in the critical care setting, and showed that the overall pooled random-effects bias was 1.50 mmHg lower for MAP measurements from NIBP. In clinical practice, a new monitoring technique is useful if its efficiency is comparable to that of the standard method. According to reference standards [22], differences of ± 5 mmHg or less for mean values and ± 8 mmHg for standard deviation between the new technique and the reference method are acceptable in NIBP measurements. The goal of this systematic review and meta-analysis, however, was to provide an estimate of the accuracy and precision of oscillometric NIBP monitoring systems to inform clinicians about what should be expected from these devices in clinical practice, especially in scenarios where IAP cannulation and measurement are not possible. However, in this case, the limits of agreement found in the studies restricted its applicability in clinical practice, precisely in clinical scenarios in which frequent and careful assessment of blood pressure is necessary, such as during the use of vasopressors [23]. Additionally, Bland-Altman analysis, the methodology performed in the studies that compose this systematic review [15–21], does not provide information on trending ability. More important than measuring MAP alone is measuring it repeatedly, according to frequent changes in clinical status [24]. In future studies exploring this topic, we need more consistent reporting of trending analysis, providing data likely to be more applicable in clinical practice [23]. In this study, we were unable to compare this variability in repeated measurements, which would have led to a greater applicability of our results.
Our study has the merit of evaluating intermittent measurement through oscillometry, which is the most widely used method of NIBP measurement in intensive care [16]. Previous systematic reviews have exclusively addressed continuous methods of NIBP measurement, with similar limitations due to the wide limits of agreement of these NIBP measurement methods when compared to IAP measurement [8, 25]. To our knowledge, this is the first systematic review exploring data on oscillometric intermittent mode. The current assessment exclusively in critically ill patients is justified because this population is more susceptible to several conditions that can, at least theoretically, influence the measurement of blood pressure [26, 27]. An estimate of effects that encompasses different scenarios increases the applicability of the results obtained, which justifies our analysis.
MAP measurement is the most commonly used blood pressure measurement in critically ill patients, according to different guidelines and recommendations in the literature [2]. The different noninvasive methods are expected to have greater accuracy in this measurement because they are methodologies designed for MAP, with the measurement of SAP and DAP derived from the first, according to the specific algorithm of each piece of equipment [9, 26, 28]. In clinical contexts where systolic or diastolic blood pressure measurements are justified as therapeutic targets, such as in hypertensive disorders, these concepts must be present, and a more liberal use of IAP may be necessary [28].
The different original studies included in this systematic review explored distinct clinical scenarios, with variable doses of vasopressors (mainly noradrenaline) and inotropes, in patients with and without arrythmia, in severe peripartum hypertensive disorders, and in different shock states [15–21]. In each study, some of these variables did not seem to influence the accuracy of NIBP measurement, such as the vasopressor dose, or even the need for high doses of noradrenaline [16], and the presence or absence of circulatory failure [17]. On the other hand, some characteristics can influence the accuracy of NIBP measurement, such as the presence of arrhythmia, the type of arrhythmia, the patient’s sex, and the BMI of the population studied [29]. With current data, we can only offer a global estimate of accuracy, lacking, in current studies, a granularity of the data. In later studies, the impact of different clinical characteristics on the accuracy of measurement should be investigated.
A major limitation of our study is the high hete-rogeneity shown in all analyses performed, which may, at least in theory, limit the applicability of the central accuracy estimate. Different clinical contexts were not analysed separately, due to the lack of data that provide a more accurate estimate [29–31]. Given the differences in the proprietary algorithms used by the oscillometric devices, comparison between them and standardization can be challenging [28], and a comparison of the accuracy between oscillome-tric and continuous NIBP measurements cannot be made [6]. Additionally, we only performed a pooled analysis of the bias. Few studies have reported the accuracy of oscillometric NIBP measurements in detecting hypotension [16, 18], which may limit the applicability of these results. Hypotension is an event associated with negative outcomes in critically ill patients [32, 33]. Although the studies described in this review have an adequate capacity to predict hypotension, further studies might provide more robustness to these initial data, as previously suggested [6].
We need further studies in this area, addressing a heterogeneous population of critically ill patients, and providing answers applicable to different patients according to their clinical and demographic characteristics. In addition, we need a better estimate of the accuracy of the oscillometric NIBP measurement in detecting hypotension, as well as its accuracy in predicting the trend (increase or decrease) of MAP according to the clinical management of the patient.