Radical tumour resection can allow a satisfactory long-term outcome, a long life without tumour recurrence, and a shorter postoperative reconvalescence. Multiparameter intraoperative monitoring, including neuronal potentials, and clinical and psychological intraoperative evaluation of the patient, optimizes the postoperative neurological outcome [1–3]. However, radical tumour resection of eloquent brain tumours is associated with the risk of motor, speech, or memory disorders due to the possible injury of an eloquent brain network [4, 5]. It is noteworthy that the depth of anaesthesia can also alter the interpretation of monitored evoked potentials and may influence high brain function [6, 7]. Neuromonitoring, such as motor-evoked potential, somatosensory-evoked potential, or neuro-navigation, plays an important role during these procedures. However, more evidence shows that conscious sedation (CS) aimed at avoiding opioids and propofol, but based on low doses of dexmedetomidine, together with neuromonitoring and intraoperative psychological or psychiatric evaluation, significantly increases the chance of a good neurological and cognitive outcome [8].
Awake craniotomy (AC) is the gold standard for surgical removal of tumours in eloquent areas of the brain. Maximal tumour resection with simultaneous preservation of brain functions such as movement, speech, and cognitive abilities are the goals of these procedures [9]. The joint use of brain-evoked potentials, neuropsychological tests, and intraoperative real-time imaging (magnetic resonance imaging [MRI], ultrasound, or computed tomography [CT]) increases the probability of maximal and functionally safe tumour resection [10–12]. However, the best method of anaesthesia for AC is still in question.
Various anaesthetic management protocols are used for AC, such as the asleep-awake-asleep technique (AAA), monitored anaesthetic care (MAC), and CS with propofol, remifentanil, and infiltration anaes-thesia [9, 10, 13, 14]. Most clinicians use the AAA technique with instrumental upper airway support during the initial and closing phase. The patient is awake only during neurological monitoring [15]. The intubation tube or the laryngeal mask insertion can be very difficult due to the patient’s fixed head position in a Mayfield clamp and the surgical dressings that cover the head. During intubation or extubation, various adverse effects can occur, such as prolonged apnoea, hypoventilation, and retention of CO2. All of these events can cause extensive brain oedema, epileptic episodes, additional brain ischaemia near the edge of the craniotomy, or intense bleeding in the operating field and may even prevent further surgery. Sedation with propofol and opioids may lead to respiratory depression with hypercapnia, and hypoxia, which results in brain oedema. Furthermore, other adverse events can occur during conventional sedation: vomiting, myo-clonus, uncontrolled movement of the patient, and non-cooperativeness [16-18].
Due to difficulties with AAA (agitation, difficult airways, uncooperativeness, sudden disagreement about being awake during surgery), another anaesthesia protocol that is better tolerated is needed. Dexmedetomidine, which is used as a basic sedative for AC, may be a promising solution. This alpha2 adrenoceptor (a2-AR) agonist works as a sedative, light anxiolytic, and analgesic through the activation of a2 brain receptors. It does not depress respiration and has no impact on the neurophysio-logy of the brain. In addition, it does not negatively influence monitored brain-evoked potentials and can protect the brain. Through activation of receptors located in the coeruleus locus area of the brain, dexmedetomidine mimics the natural physiological pattern of sleep without REM phase II/III [11–13].
In this study, the results of a CS research protocol using dexmedetomidine infusion and a scalp block with ropivacaine are presented. The objective of this research was to evaluate the risk of respiratory and circulatory complications, changes in neurophysiological monitoring, and other adverse events during AC. The vital element of this protocol was the psychological evaluation before, during, and after surgery.
METHODS
Study design
This study was a single-centre prospective study without a control group for comparison, approved by the Bioethics Committee of the University Hospital in Wroclaw (10.10.2013) and by the Bioethics Committee of the Wrocław Medical University (KB – 279/2016 [02.06.2016]) in accordance with the Declaration of Helsinki. Written informed consent was obtained from all subjects.
Thirty-one patients admitted to the University Hospital in Wroclaw between 2014 and 2018 were screened to ensure they met the study inclusion criteria. The main inclusion criteria were a single brain tumour localized near or in the eloquent cortical areas (qualified for elective AC), good neurological state, age > 16 years, ASA 1–4, good patient cooperation, and good intellectual function confirmed by neuropsychological tests. Exclusion criteria included alcohol or drug abuse, psychiatric disorders, severe anxiety, allergies to study medications, recent clinical manifestation of epilepsy, electrolyte or acid-base imbalance, significant glucose imbalance, low heart rate (< 55 bpm), arrhythmias and lack of neuropsychological evaluation. Figure 1 presents a flow chart showing exclusion criteria and the experimental design of the study. Ultimately, 26 patients were enrolled in the study.
Neuropsychological assessment
The preoperative patient evaluation was performed at least one day before surgery. A neuropsychological evaluation, including a general assessment of neurological and psychological status, as well as an evaluation of the higher mental functions (such as speech, memory, thinking, perception, and attention), was performed using the following battery of tests (before surgery): STAI – Inventory of State and Anxiety (C.D. Spielberger, R.L. Gorsuch, R.E. Lushene), Eysenck Personality Questionnaire-Revised Short Scale (EPQR-s), Hamilton Depression Rating Scale HAM-D, Numerical Rating Scale, Benton Visual Retention Test, Hodges J. Addenbrooke Cognitive Examination – ACR-III, Schmidt M. Rey Auditory Verbal Learning Test (RAVL); (intraoperatively): Hodges J. Addenbrooke Cognitive Examination –ACR-III, and W. Łucki questionnaire for the study of cognitive processes in patients with brain injuries. Furthermore, each patient was informed of the operation in terms of decision-making and motivation for participation. The issue of stress management, anxiety, and claustrophobia was also discussed. Patient treatment satisfaction was assessed using the Postoperative Short Psychological Questionnaire (see Supplemental File) and the following battery of tests: Addenbrooke’s Cognitive Examination – ACE-III, Rey’s Auditory Verbal Learning Test (RAVL), Benton’s Visual Retention Test, and the numerical rating scale.
Anaesthesia procedure, intraoperative monitoring
Premedications were not used, to avoid any possible influence on cognition. We avoided a loading dose of dexmedetomidine due to the risk of cardiovascular depression. We prefer a stable low-dose infusion of dexmedetomidine (0.1–1.0 µg kg–1 h–1). The level of sedation was adjusted to the surgery phase to reach a level of sedation of –1/–3 on the Richmond Agitation Sedation Scale (RAAS).
After intraarterial cannulation under local anaesthesia, the following parameters were monitored: electrocardiography, heart rate (HR), intraarterial blood pressure, bispectral index monitoring (BIS) (Integra, Ohio, USA) with electrodes typically fixed on the contralateral side of the craniotomy, saturation (Pulsoximetry, LG, Korea), respiratory frequency, and EtCO2 with a nasal cannula. Saturation, EtCO2, systolic arterial pressure (SAP), diastolic arterial pressure (DAP), mean arterial pressure (MAP), and HR were collected and analysed at baseline before dexmedetomidine infusion (T0), and then 5, 10, 30, and 60 minutes (T5, T10, T30, T60, respectively) after the start of dexmedetomidine infusion and 60 minutes after discontinuation of dexmedetomidine (Tpo). Arterial blood gases (PaCO2, PaO2), electrolytes, and glucose levels were collected and analysed at T0, T30, T60, and Tpo. Furthermore, a modified Bristow Scale was used to assess brain relaxation after dura opening (quality of operative condition).
The standard analgesia plan was provided perioperatively to our patients. Paracetamol (acetaminophen) 1.0 g IV or metamizole 2.5 g IV was given before skin incision, and then both drugs – postope-ratively, in dose 1.0 g IV every 6 hours and 8 hours, respectively. It was continued for 24–48 hours according to the requirements of individual patients. There were no patients with an allergy to those drugs in the presented cohort of patients. A scalp block was performed using 0.75% ropivacaine with adrenaline 1 : 200,000. If a patient complained of pain, assessed on the Visual Analogue Scale (VAS), then additionally IV/SC oxycodone was used at a dose of 5–10 mg every 4 hours.
The small doses of propofol (10–20 mg IV) were used for scalp block, head fixation with a Mayfield clamp to the meningeal opening, and skull closure. Some patients were also given small doses of midazolam (0.5–1.0 mg IV) after the neuromonitoring and psychological evaluation phase. Before skull pin application, local skin was anaesthetized with 2–3 mL of 1% lidocaine and adrenaline 1 : 200,000 at each pin site. Next, each patient received ondansetron (8 mg IV), omeprazole (40 mg IV), dexamethasone (8–12 mg IV), and mannitol (0.5 to 1.0 g kg–1) within 20 to 30 minutes of skin incision. The infusion was completed before opening the dura. The dura was then flushed with 10 mL of a 1% lidocaine solution before the incision. In the event of an epileptic seizure, the following medications were prepared: clonazepam, valproate acid, midazolam, propofol, and ice-cold saline (to apply to the operating field).
Oxygen was provided through a mask at 2–4 L min–1, and PaO2 was maintained at a level between 100 and 160 mmHg. Instrumental airway management and conversion to general anaesthesia were prepared (if necessary). The patient was placed according to the surgeon’s preference and checked to ensure safe positioning. The neck and legs were supported with pillows as needed. The patient’s face was uncovered with surgical covers secured above the face. Constant and clear access to the head was maintained for patient observation and to allow instrumental airway support if needed. Before mapping the cortex and neurological tests, the infusion of dexmedetomidine was stopped. During the post-monitoring closure phase, the sedation was deepened, if necessary, with an infusion of small doses of propofol, midazolam, or fentanyl.
Neurophysiological monitoring
During surgery, the following brain potentials and neurophysiological tests were performed: motor-evoked potentials (MEP) – after direct electrical stimulation of the cortical and subcortical brain regions, brainstem-evoked auditory potentials (BEAP) – for the evaluation of brain function, sensation – assessed by a subjective feeling of sensory phenome-na by the patient. Motor functions were evaluated based on electromyography (EMG) traces. During the resection of the brain tumour, the patients performed motor tasks to engage specific groups of muscles necessary for the movement of the indicated limb. Furthermore, the patients contracted the indicated muscles while needle electrodes were inserted. The higher psychological functions, such as speech, memory, attention, and perception, were assessed with neuropsychological tests. The entire exposed gyri were stimulated directly in 5-mm steps using a bipolar electrode with a tip distance of 5 mm. During stimulation, patients were asked to perform either a counting task or a naming test. All black and white pictures presented were familiar to the patient according to preoperative assessment, and each name was preceded by ‘this is’ [16]. Speech arrest or the appearance of semantic errors was considered a positive result of mapping and led the surgeon to mark it [17].
Statistical analysis
Statistical analyses were performed with Statistica software (v.13.1, StatSoft Inc.). Descriptive statistics are presented as mean ± SD unless otherwise indicated. Statistical significance was assumed at a = 0.05. Data distributions were tested using a Kolmogorov-Smirnov test. The hypothesis of normality was rejected for most of the analysed parameters; therefore, nonparametric tests were used. To evaluate the differences between physiological parameters at baseline before dexmedetomidine infusion (T0) and then 5, 10, 30, and 60 minutes (T5, T10, T30, T60, respectively) after beginning the dexmedetomidine infusion and 60 minutes after discontinuation of dexmedetomidine (Tpo), a Friedman nonparametric ANOVA test was applied. Post-hoc analyses were performed using Wilcoxon signed-rank tests with Holm-Bonferroni corrections for multiple comparisons. The Spearman rank correlation coefficient was used to examine the linear relationships between BIS and RASS.
RESULTS
Patients’ characteristics and procedure outcomes
The group consisted of 16 men and 10 women, with a mean age of 51 ± 17 years (min 19 years, max 77 years). The clinical characteristics of the patients are presented in Table 1. The mean surgery time was 6 h 10 min ± 1 h 25 min. The consumption of dexmedetomidine during the procedure was on average 711 ± 240 µg (min 400 µg, max 1200 µg). The application of other drugs was marginal in the total group: propofol 36 ± 51 mg (max 150 mg), fentanyl 0.01 ± 0.03 mg (max 0.10 mg), and midazolam 0.08 ± 0.27 mg (max 1 mg). No catecholamines were used intraoperatively. Small doses of ephedrine were administered to 3 patients for transient blood pressure drops. Significant (> 500 mL) intraoperative blood loss was noted in one patient (4%) with a large meningioma.
TABLE 1
Demographic and clinical data in the total group (N = 26) of patients who underwent awake craniotomy for tumour removal
Intraoperative brain relaxation assessed using the modified Bristow scale was assessed as 2 points (a compliant brain within craniotomy edge) in 22 patients (84%), as 3 points (slightly tight brain, little above craniotomy edge) in 3 patients (12%), and as 4 points (tense brain, brain oedema, significantly above craniotomy edge) in one patient (4%). Surgical conditions were excellent in 6 cases (23%), good in 16 cases (62%), and poor in 4 cases (15%; all with intraoperative epileptic episodes).
Intraoperative and postoperative complications
Intraoperative and postoperative complications are presented in Table 2. Intraoperative epileptic episodes were observed in 4 patients (15%). The patients experienced generalized seizures caused by electrical stimulation of the motor cortex (EEG monitoring). The seizures were observed in 31% of patients with an identified motor cortex. The onset of seizures in 75% occurred during electrical stimulation of the cerebral cortex. However, in one case, a generalized tonic-clonic seizure occurred during traction placed on brain tissue or the use of a Cavitron Ultrasonic Surgical Aspirator (CUSA). All patients who experienced seizures during surgery presented transient lower limb muscle force. In one case, decreased consciousness without accompanying seizure was observed during motor cortex stimulation. None of them showed sensory or speech disorders; however, no eloquent areas were identified in these patients. In the event of a seizure, the surgical field was filled with ice-cold Ringer’s solution, and in half of the cases, an additional 1 mg of clonazepam was administered intravenously, resulting in regression of convulsive activity and restoration of consciousness.
TABLE 2
Operative conditions, intraoperative complications, and clinical results in the total group (N = 26) of patients who underwent awake craniotomy for tumour removal
The following abnormalities were found on postoperative head CT (24 hours after surgery): haematoma in 3 patients (12%) and venous stroke in one patient (4%). No reoperation was needed. Except for one ASA-4 patient, no patient required ICU observation. In this case, the patient had a severe chronic obstructive pulmonary disease and pulmonary tumours compressing the aorta that required transfer to the ICU for 24 hours of observation. Postoperative follow-up was good in all patients (including the ASA-4 case). A death in the late postoperative period (7th day after AC) was caused by a pulmonary embolism.
Intraoperative monitoring
SAP, DAP, and MAP changed significantly during the analysed points in time (χ2 = 45.40, P < 0.001; χ2 = 33.56, P < 0.001; χ2 = 21.94, P < 0.001, respectively). These alterations were within the clinically accepted range during the measurements and did not exceed 20% of the initial values. SAP was lower at T30 (P = 0.001), T60 (P < 0.001), and Tpo (P < 0.001) vs. T0. DAP decreased at T30 (P = 0.002), T60 (P < 0.001), and Tpo (P < 0.001) vs. T0. MAP decreased at T60 (P = 0.001) vs. T0 (Figure 2A). HR altered significantly at the analysed points of time (χ2 = 34.20, P < 0.001). HR decreased at Tpo vs. T0 (P = 0.001) (Figure 2A). Those changes were unimportant (20% change from the initial value).
FIGURE 2
The median values and interquartile ranges (boxes) and min-max values (whiskers) of (A) mean arterial pressure (MAP) and heart rate (HR) (B) blood gas concentrations: PaCO2 and PaO2 changes at the monitored time intervals: at baseline before dexmedetomidine infusion (T0), then 5, 10, 30, and 60 minutes from dexmedetomidine infusion start (T5, T10, T30, T60) and 60 minutes after dexmedetomidine discontinuation (Tpo); comparisons between T0 and following time intervals were tested using a post-hoc Wilcoxon signed-rank test
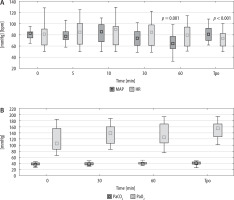
The saturation did not change significantly at the analysed points in time, and at no point in time was it less than 94%. EtCO2 did not change considerably during the analysed points of time. PaCO2 and PaO2 increased over time (χ2 = 14.38, P = 0.002; χ2 = 9.70, P = 0.021); however, neither parameter reached statistical significance in post-hoc tests compared to T0 (Figure 2B). The values remained within the normal range, so the increases did not have a significant impact on brain compliance or operative conditions. The range of BIS was 50 to 98 and was lower at T10 (P = 0.002), T30 (P < 0.001), and T60 (P < 0.001) vs. T0. The BIS changes followed the RASS changes (rs = 0.40; P < 0.001).
Neuropsychological monitoring
Most patients perceived AC as a positive (50%) or neutral (27%) experience (see ‘Postoperative short psychological questionnaire’, question 1 and Figure 3). 18% of patients assessed AC as a negative experience, and 6% of patients would not undergo awake surgery again (see ‘Postoperative Short Psychological Questionnaire’, question 4). The post-operative interviews with patients showed that the greatest sources of discomfort and pain were clamping, operating position, limited field of vision, and staff behaviour, which can lead to iatrogenic disorders. Factors that patients mentioned as a source of support (see ‘Postoperative Short Psychological Questionnaire’, Question 2) were as follows: extensive communication, a relaxed atmosphere, feedback from an operator (especially positive), attendance of a neurophysiologist and psychologist, and mental preparation before operation by members of a specialized team (operator-neurosurgeon, attending physician, anaesthesiologist, neurophysio-logist, psychologist).
The mapping of the eloquent areas of the brain cortex was performed based on correct word articulation (aloud counting from 1 to 10) and the name of the presented images in 81% of the cases. During the stimulation of the cerebral cortex, only 3 patients suffered speech deterioration. Two of them had a minor speech deficit of 1/2 intensity, and in one case, the speech deteriorated. In this single case, partial resection of the left cingulate gyrus resulted in akinetic mutism – speech disorder in grade 0/2, which improved after logopaedic therapy in the postoperative period. Patients who had intraoperative seizure attacks did not show any speech disorders.
Intraoperative neurophysiological monitoring
Intraoperative neurophysiological monitoring was performed in 96% of AC patients. Electrical mapping of the cerebral cortex allowed the identification of the motor cortex in 50% of patients and the subcortical structures in 12% of the cases. Preoperatively, muscle force was 5/5 in 85% of patients and 4/5 in 2 patients, using the Lovett scale. Unaltered muscle force was observed postoperatively in 88% of the patients. Postoperatively, one patient decreased from 5 to 4 on the Lovett scale and 2 others from 5 to 1. Additionally, one patient with a score of 4/5 was temporarily paralyzed after surgery. Sensory function measured after cortex or peripheral stimulation was evaluated in 62% of patients, and in 22% of cases the sensory cortex was successfully stimulated. None of the operated patients reported quantitative or qualitative disturbances during surgery. Brainstem auditory-evoked potentials were recorded in 15% of patients. The latency and amplitude of peaks I–IV, II, III, and V, as well as the interpeak latency of I–III and III-V, were close to the initial values. Of all patients eligible for AC, 73% had a previous diagnosis of epilepsy. Suppressive treatment in 74% of the patients required an antiepileptic drug, and 26% required 2 different drugs.
DISCUSSION
AC is a technique developed for maximizing resection while preserving neurological function [1]. Multiple studies have documented that CS based on low doses of dexmedetomidine, along with neuro-monitoring, is a feasible approach [18–20]. Dexmedetomidine-based CS with a scalp block without instrumental airway support in conjunction with psychologist support provides good quality intraoperative sedation for AC, even during long surgical procedures requiring intraoperative magnetic resonance imaging. The protocol of anaesthetic management proposed in this study allows one to achieve a good quality of analgesia while still maintaining sedation with fast recovery to full consciousness for neuropsychological monitoring after cessation of dexmedetomidine infusion. It is comfortable and not too demanding for the patient. The clinical study showed that the anaesthe-tics used in this protocol did not significantly influence cardiovascular and respiratory parameters. Operative conditions were good according to the surgeon’s opinion and the modified Bristow scale. The quality of the monitored brain potentials, as well as neuropsychological monitoring, was satisfactory. We did not observe symptoms of cough, nausea, vomiting, agitation, anxiety, brain swelling, or haemostatic disorders intraoperatively, as reported in the literature [18, 21].
Various anaesthetic management strategies for AC are being utilized; however, there are no clear recommendations [14]. Several authors have researched propofol-remifentanil and dexmedetomidine-based sedation, but only a few comparative studies have been performed [22–24]. Dexmedetomidine has been reported to depress the cardiovascular system by relaxing the autonomic system, increasing the risk of global or regional hypoperfusion of the brain [25, 26]. However, in our study, no clinically significant influence on cardiovascular or respiratory parameters was observed. SBP, DBP, MAP, and HR decreased significantly at T30 and T60 compared to initial values and continued at that level until 60 min after discontinuation of sedation. It is noteworthy that these changes did not reach clinical significance. Similar results were reported in another retrospective observation on a large cohort of patients, where the doses of dexmedetomidine used in that trial (up to 1.7 µg kg–1 h–1) were higher than what was proposed in this study [21]. However, due to the limited group of patients in our study, the impact of dexmedetomidine on the cardiovascular system requires further investigation.
We also marked that, except for one case, patients were not admitted to the intensive care unit, due to stable immediate postoperative status. After AC, patients were taken to a postoperative recovery room for 2–3 hours and then transferred to the neurosurgery unit. They were allowed to drink a few hours later. The next day, they were mobilized after the completion of the postoperative head CT. The duration of hospitalization was as short as 48 to 72 hours in 2 patients. The remaining patients required longer hospitalizations due to neurological dysfunctions or fear of delayed new neurological complications and inadequate supervision after being sent home. It is noteworthy that because of the short patient hospitalization after AC, the cost of the entire procedure would be greatly reduced compared to general anaesthesia [27, 28].
In this study protocol, a sedation level of –1/–3 on the RASS scale was maintained up to meningeal closure, and tests were frequently performed to warn the operator of possible white matter injury that could cause further emotional, memory, or character disorders [29]. The level of sedation was also monitored with BIS. It is well documented that the RASS scale and BIS are correlated with the level of sedation [30, 31]. Such a correlation was also found in this study. As reported previously [32], we did not observe a significant change in BIS values under sedation with dexmedetomidine RASS –1/–2, but we observed lower BIS values after a bolus of propofol and initiating deeper anaesthesia (RASS –3). The time to achieve complete consciousness for a patient was 3–5 min (from RASS –1/–2), up to 10–13 min (RASS: –3) after stopping dexmedetomidine, and is consistent with other research. This study showed that RASS –2/–3, corresponding to BIS 98–50, is comfortable for the patient while allowing them to regain complete cooperativeness in a few minutes at any time during surgery to allow neuro and psychological evaluation.
The risk of seizures triggered by electrical brain stimulation is a common adverse event during brain mapping. This study has shown that dexmedetomidine does not influence epileptiform discharges in EEG after IV infusion [33]. In cases without EEG, continuous free-running EMG recordings during the surgical removal procedure were successfully used to identify increased EMG activity and possible imminent seizure. The 15% incidence of intraoperative seizures experienced in our study was within the range described in the literature [34]. The seizures were predominantly caused by relatively high- intensity stimulation, which usually exceeds 10 mA despite the use of 50-Hz stimulation. In 3 cases the seizures were generalized, and in one case it was a focal seizure, and all were stopped with irrigation with Ringer solution. Half of the patients who experienced seizures had motor deterioration, but the motor functions of the remaining patients were stable despite preoperative weakness.
The quality of the monitored brain potentials did not change from the initial value. It was pre-viously shown that appropriate dosing of dexmedetomidine is crucial to avoid its suppressive effect on motor function. The motor function changes observed in our patients were a consequence of tumour removal manoeuvres rather than a direct pharmacological influence on cerebral blood flow that affected motor function [35]. Patients who regu-larly performed simple and complex motor tasks to assess agility and muscle strength were well tolerated by them and did not alter the progress of the operation. The results obtained from motor function monitoring were informative for the surgeon and anaesthesiologist and consistent with the postoperative neurological achievements of the patients [36]. Intraoperatively and postoperatively, all patients did not complain of sensory disturbance.
The indications for the use of CS for AC are increasing: all grade 1–2 WHO gliomas, basilar artery aneurysms, and other complex aneurysms, deep brain stimulation, cerebellopontine tumours, and patients with complex comorbidities and obesity [29]. Furthermore, according to recent recommendations, some of the patients included in this study would have been disqualified from using the AAA technique or any other type of CS for AC using propofol, fentanyl, or remifentanil due to severe obesity, difficult airways, and severe respiratory and cardiovascular comorbidities due to an unacceptable increase in risks of severe intraoperative complications. Also, most anaesthetists would have excluded some of the surgical positions (park bench, sitting, prone) if a different type of sedation were used. Patients with severe comorbidities or obesity are the best candidates for the techniques presented in this study becuase many complications can be avoided.
Neuropsychological examination, which was not affected by dexmedetomidine, allowed efficient protection of language and cognitive skills. It was shown that dexmedetomidine did not block differentiation between congruent and incongruent language tasks based on the presence of N400 and is recommended during craniotomy [37]. It is worth mentioning that 50% of the patients had language disturbances before surgery, but only 30% of the electrical mappings allowed the identification of cortical language epicentres. After the operation, deterioration of the language was observed in 4 cases (15%).
The preoperative psychological evaluation was an important part of our study. The patients in our study were evaluated by the psychologist not only before AC but also during the total procedure and 24–48 hours after AC. The presence of a psychologist during the whole procedure (in the operating room) is not a standard element of the procedure. Previous studies have shown that hypnosis could be used successfully in the paediatric population during AC for the resection of brain tumours located close to eloquent areas [38] and during AC of low-grade gliomas [39]. Thus, we expected that, similarly to hypnosis, the presence of a psychologist would encourage patients to gain motivation and safety during AC and decrease the risk of conversion to general anaesthesia. Our experience indicates that building patient trust and comfort can increase the chances of a positive patient experience during the long-lasting procedure and after hospital discharge. The detailed psychological evaluation of patients in terms of early and late postoperative stress injury will be a matter of a separate report.
The age distribution in our group of patients was as follows: one case was < 20 years, 7 cases were between 20 and 40 years, 14 cases were between 40 and 65 years, and 4 cases were > 65 years. The reactivity of sedative-analgesic agents could depend on age [40, 41]. However, the Kruskal-Wallis ANOVA test did not show significant differences in the total dose of dexmedetomidine (c2 = 2.65; P = 0.265), which is in line with other studies on the pharmacodynamics and pharmacokinetics of dexmedetomidine [42].
The anaesthetic protocol during AC is still under investigation. Abazieu [43] reported that remifentanil could be an independent risk factor for adverse events during AC. Remifentanil can depress the respiratory centre in the brain and cause nausea and vomiting, leading to respiratory insufficiency, prolonged hypoventilation, and conversion to general anaesthesia. It should be noted that a combination of this opioid with propofol is generally recommended and used (including in the author’s centre) for the AAA protocol of anaesthesia [43]. This sedation procedure probably should be questioned, especially if we recognize that already impaired neuronal function in operated patients, due to any further imbalance, might be irreversibly damaged. It has been shown that in older patients anaesthetized with remifentanil and propofol, there were higher incidences of cognitive deterioration. Such mental disorders were not observed in patients sedated with dexmedetomidine and propofol [44]. Furthermore, the other authors underlined the negative impact of propofol on cognitive functions, which can mask real neuronal dysfunction and cognitive impairment that must be minimized during AC [45].
This observational clinical study has limitations. The procedures performed were long (about 5 hours on average), which can affect the patient’s comfort. However, dexmedetomidine-based sedation led to physiologically similar sleep with immediate “on- demand” arousal. Other studies on dexmedetomidine sedation have analysed much shorter procedures in retrospective research, which could have an impact on the conclusions [25]. Secondly, we only analysed clinical parameters a few moments from the start of dexmedetomidine infusion to 60 minutes after infusion. However, we noticed that all monitored parameters were stable from T60 until the end of the surgery. Third, although anaesthetic management was similar for all analysed patients, some subjects required a bolus of propofol or mida-zolam during head closure to maintain patient comfort. The synergistic effect of these chosen supplements deepens anaesthesia without the risk of adverse events. In addition, the relaxation of the brain and the operative conditions were based on the observation of an experienced neurosurgeon. The modified Bristow scale seems to be a suitable tool to access for brain relaxation assessment, but it is subjective. Lastly, the character of this study was prospective without a control group. However, the lack of adequate control was not perceived as a major deficiency in the study design. This research has been designed as uncontrolled due to previous experiences in the AAA procedure that propofol and opioid sedation led to respiratory depression, uncontrolled movements, poor arousal quality, long recovery to cooperative consciousness, and high risk of respiratory complications during AC. Furthermore, the number of patients who met the inclusion criteria for AC was minimal at that time in our centre. Given these limitations, a multicentre study with a control group would provide more reliable data and improve the generalizability of these observations.
CONCLUSIONS
CS with dexmedetomidine and scalp block can provide a safe and comfortable anaesthesia procedure during AC for the surgeon and the patient. It allows the implementation of advanced, multilevel intellectual tests, where perioperative psychological support plays an important role. Smooth induction and arousal with a cooperative patient are possible with the presented anaesthetic method, even during long-lasting procedures (5–6 hours). Further observations and comparative trials are needed to conclude the best anaesthetic protocol for AC procedures.