Purpose
Although surgical resection represents the gold standard, in the past decade there was an expansion in the use of non-surgical ablative techniques in primary as well as secondary liver cancers. With the growing experience in the field, the importance of multidisciplinary teams of specialized centers was realized in an optimal treatment procedure, implementing the so-called interventional oncology (IO).
Due to the close cooperation between interventional radiology and interventional radiotherapy (brachytherapy) experts, beside widely accessible 3D-conformal or stereotactic external beam radiation [1,2,3], local non-surgical interventional tumor tissue ablation represents nowadays an additional option for the treatment of patients with cancer, together with surgery, chemotherapy, and radiotherapy. Few years ago, percutaneous interventional ablation techniques were exclusively, indicated as palliative and experimental treatments [4]. However, today the scenario has completed changed, with ablation treatments also used as a first-line option in selected tumors and selected patients [5,6]. These treatments are also suitable for nonsurgical well-selected patients, with technical or clinical restrictions, based on multidisciplinary tumor board decision [7,8,9,10]. Furthermore, in some cases IO treatments are also preferred from a cost-effective point of view [11]. Radiation therapy is preferably performed in most of the institutions as external beam stereotactic radiotherapy [12], and high levels of evidence do exist in different primary tumor anatomic sites and stating the survival advantage of treating oligometastatic disease, if the primary is stable [13,14]. However, interstitial computed tomography (CT) guided implantation and irradiation has been described as an appropriate and successful method of local radiation [15]. However, percutaneous liver stereotactic body radiotherapy (SBRT) is a successful method in treating liver targets [16]. It is assumed that a rapidly increasing future workload of external beam facilities will allow an optimal patient service, and spare valuable external beam facility time [17].
Additionally, the use of brachytherapy can reduce the number of treatments needed, resulting in fewer visits to the radiotherapy department, potentially increasing the number of patients recruited [18]. Also the need for dose escalation as a biologically equivalent dose (BED) of 100 and possibly higher in some histologies is needed for control, which can easily be achieved with brachytherapy in the case of multiple targets or following previous radiation treatments [19].
There is a lack of comparative interdisciplinary evaluation of all these non-surgical interventional local ablation methods. The present paper would like to offer a critical interdisciplinary overview of the treatments in both primary as well as secondary liver tumors, including from a cost-effectiveness point of view.
Percutaneous ablation treatments
Ablation technology has rapidly evolved during the past few years, with substantial technical and procedural improvements that can help to increase clinical outcomes and safety profiles. The future of ablation should be based on an attempt to expand clinical indications as appropriate, creating the potential for larger areas of ablation, attempting to more precisely control the area of ablation, ensuring the safety of the procedure, and achieving even greater long-term success in terms of complete response rate, progression-free survival, and overall survival [20]. Recent developments include several new techniques to improve treatment efficacy. Within the classification of tumor ablation, several modalities are used worldwide: radiofrequency, microwave, laser, cryoablation, and irreversible electroporation. In detail, thermal energy is used to heat (radiofrequency, microwave, laser) or cool (cryoablation) tissue to cytotoxic levels (more than 60°C or less than −40°C). Alternative non-thermal technique is represented by irreversible electroporation, which targets the permeability of the cell membrane. Most ablation systems are based on a generator and a needlelike device that delivers the energy directly to the targeted tissue to achieve acute cellular necrosis. Each technique, although similar in purpose, has specific and optimal indications, with different advantages and disadvantages, which need to be briefly discussed.
Radiofrequency ablation
Radiofrequency ablation (RFA) is considered the first-line ablation technique, with advantages and limitations well demonstrated in experimental animal studies and clinical trials. It is based on low frequency radio waves with a long wavelength to determine heat, by ionic agitation with the adjacent molecules closer to the applicator, resulting in frictional indirect heating, with consequent coagulative necrosis and cell death [21,22,23]. RFA is characterized by a limited necrotic volume provided, eventually requiring multiple probes for lesions larger than 3 cm in size, with limitations mainly related to the heat sink effect as well as the risk for tumor carbonization, based on excessive desiccation of tissues which increases impedance and decreases the electrical current flow. Specific advantages are represented by well-proved safety and reproducibility/procedural standardization, with optimal results for primary and secondary hepatic tumors up to 3 cm in size; it is also characterized by a simple technique, low-profile/minimal-invasive ablative probe, as well as low cost of a single needle. The multiple probe approach can be used in order to treat larger lesions [24,25,26,27,28,29]. Major complications of RFA include intraperitoneal bleeding, infections, liver failure, pneumothorax, organ injury, bile duct stenosis and tumor lysis syndrome; however, major complication and procedural mortality rates are significantly low [30,31].
Microwave ablation
Microwave ablation (MWA) is becoming a competitor to RFA; its mechanism of action is based on rotation or oscillation of water particles, resulting in direct heating. It is not limited by carbonization, with consequent faster heating and higher temperature available, with better propagation of electromagnetic waves. It is less influenced by tissue properties and not influenced by the heat sink effect. Finally, the increased thermal conductivity combined with increased energy deposition potentially create larger coagulation necrosis with potential better clinical results in terms of the local effect obtained. Despite the theoretical advantages from these improvements in MWA, to date, it remains unclear whether these are associated with actual clinical benefits in outcome. Randomized trials and meta-analyses showed equivalent therapeutic effects and complication rates between MWA and RFA ablation in the treatment of hepatocellular carcinoma (HCC) and colorectal liver metastases respectively [32,33,34,35,36,37]. This lack of agreement may be related to changes in generators/antennas used in the various studies. However, some interesting potentially beneficial features of MWA in comparison with RFA can be considered, including the shorter procedural duration and the easy placement of one straight antenna to obtain volumes of ablation that would require more than one RFA cooled electrode or expandable electrodes.
To date, MWA seems to be preferred for HCC larger than 3 cm in size or for metastatic lesions up to 4 cm in size (Figure 1). It needs to be underlined that for HCC larger than 3 cm in size it could also be used as combined treatment, performing in a single-step approach both chemoembolization and ablation, with a low complication rate and high CR rate obtained [38,39], also available in patients with complex lesions or with high risk of procedural bleeding [40].
Fig. 1
Single liver metastasis from adrenal cancer in the VIIIs, close to inferior cava vein in a 43-year-old man. Contrast enhanced CT image in axial plane (A) shows hypervascular focal lesion 2 cm in size (arrow). The patient underwent percutaneous MWA ablation of the lesion (B). Contrast-enhanced CT examinations performed 3 months (C) and 12 months (D) after the procedure demonstrate complete necrosis without residual enhancing tissue in the ablated area, also preserving patency of inferior cava vein (post-treatment complete response)
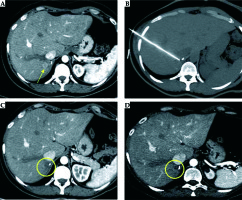
Cryoablation
Cryoablation is the only technique which uses freezing instead of heat to create tumor necrosis. Its mechanism of action is based on the Joule-Thompson effect with the use of a gas, such as argon, which rapidly decreases to subzero temperatures (as low as –150°C) upon transition from a liquid to gaseous state; the freezing is alternated with a thaw cycle, during which helium gas is administered to raise the temperature to about 40°C. The treatment protocol is composed of two 10-minutes cycles of freezing with a 5-minute break of thawing. Cell death is obtained with both direct and indirect mechanisms. In detail, rapid freezing determines the formation of intracellular/extracellular ice crystals with consequent disruption of the cell membrane and internal processes. Furthermore, indirect mechanisms include vasoconstriction and occlusion of blood vessels resulting from osmotic changes and local tissue edema resulting in hypoxic tissue injury and coagulative necrosis. Finally, they also generate immunological interactions and promotion of inflammatory cytokines, which may also exert a tumoricidal effect [41,42,43].
The main advantages of the procedure are represented by: a) the much shorter intraprocedural pain, requiring only mild sedation, in particular for lesions near the diaphragm or abdominal wall, b) the tissue preservation and healing with resistance of collagenous architecture, c) the direct intraprocedural visualization of “core necrotic volume” on CT or MRI without contrast medium, with better necrosis predictability and control. Disadvantages are represented by the limited necrotic volume obtained (maximum transverse diameter obtained: less than 2 cm in size), with the need to use a multiple probe approach, the bulkiness of equipment (gas), the longer time of the procedure (more than 30 minutes versus less than 12 minutes for RFA or MWA), and the higher cost of the device (more expensive than RFA and MWA), also considering the need of multiple probes for obtaining a volume necrosis available for the treatment of a nodule of 3-4 cm in size.
Some authors have postulated that there may be a reluctance to treat hepatic tumors with cryoablation, perhaps because of concerns for bleeding, liver fracture, and cryoshock that were observed many years ago during open cryosurgery [44]. However, these severe complications typically occurred after cryoablation of large tumors and involved the placement of applicators as large as 9 mm in diameter, much larger than those currently percutaneously used [45]. A single prospective randomized clinical trial compared percutaneous cryoablation to RFA for the treatment of HCC, obtaining a similar overall 5-year survival rate [46].
Based on the intraprocedural monitoring of ice ball formation minimizing the chance of harming adjacent critical structures, the limited ice ball extension beyond the liver into the diaphragm or body wall with less pain and injury than RFA [47], cryoablation could be preferred to RFA or MWA to treat hepatic masses close to critical structures, such as the diaphragm, chest wall, heart, lung, gallbladder, and biliary tree [48,49], with better results for lesions less than 4 cm in size [50] (Figure 2).
Fig. 2
Ovarian metastatic implants in the perihepatic visceral peritoneum mimicking hepatic metastases in a 58-year-old woman. Axial post-contrast CT image (A) shows two subserosal hepatic deposits of ovarian cancer with rounded, well-defined margins, 12 mm in size (circles). In order to obtain displacement of neighboring right kidney and duodenum, preventing untargeted ablation, a percutaneous hydrodissection using a Chiba needle (arrow) was performed (B). Thus, a simultaneous ablation of the two lesions was performed using two different cryoprobes (IceSphere, 2 freezing/thawing cycles) (C) obtaining, on 6-month follow-up contrast-enhanced CT examination (D), a complete response, without signs of residual viable tumor (arrowhead)
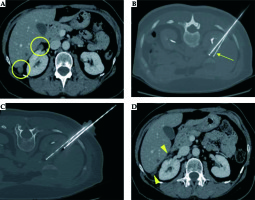
Laser interstitial thermal therapy (LITT)
With laser ablation, direct heating destroys tissue with low-power and highintensity laser light energy delivered percutaneously via thin optical fibers, with a diameter from 300 to 600 μm. Conventional bare-tip fibers provide an almost spherical thermal lesion of 12-15 mm in diameter, and a beam-splitting device or a multi-source device allow for the use of up to four fibers, simultaneously delivering the light into each single fiber.
Laser thermal ablation (LTA) technique, as proposed by Pacella et al. [51] and improved by Di Costanzo et al. [52], consists of 300 μm bare optical fibers introduced into the tumor through 21-gauge needles. The diameter of the needles is smaller than RFA electrodes and MWA antennas, making LTA safer and more suitable for ablating lesions in at-risk locations or in locations that are difficult to reach [53]. Moreover, a multisource device allows from one to four fibers to be used at once, making it possible to achieve ablation areas from one to 4-5 cm in diameter, and consequently to treat tumors ranging from 5-6 mm to 3 cm in diameter, obtaining an acceptable safety margin.
Laser thermal ablation could be proposed as a valid alternative to RFA for lesions up to 2 cm [54], and it has been suggested as the technique of choice in the presence of multiple small and variably sized liver tumors. It needs to be underlined that the efficacy of LTA can be limited by the heat-sink effect, like RFA.
Electroporation
Reversible (RE) or irreversible (IRE) electroporation is a non-chemical non-thermal image-guided ablation technique [55]. Reversible electroporation is characterized by the creation of temporary and limited pathways for molecular transport via nanopores, but after the end of the electric pulse, the transport ceases and the cells remain viable. Medical applications include, for example, local introduction of intracellular cytotoxic pharmaceuticals such as bleomycin (electroporation and electrochemotherapy).
On the other hand, IRE is characterized by a certain degree of damage to the cell membranes by electroporation.
The leakage of intracellular contents could be too severe or the resealing of the cellular membrane too slow, leaving healthy and/or cancerous cells irreversibly damaged. They die by apoptosis, which is unique to this ablation technique, in opposition to all other ablation systems, which induce necrosis either by heat, cooling, or radiation.
It is a method to induce irreversible/reversible disruption of cell membrane integrity by changing the transmembrane potential resulting in cell death without the need for additional pharmacological injury. Because IRE is potentially a non-thermal technique, issues associated with perfusion-mediated tissue cooling or heating (a significant challenge with thermal methods) are less relevant. So far, the published data of the technique are mostly represented by case series including tumors in difficult perivascular or peribiliary locations [56,57].
Devices for IRE are more expensive than other ablative needles.
Electrochemotherapy
Electrochemotherapy (ECT) is also a non-thermal ablation, similar to a chemoablation, as a chemotherapeutic agent accomplishes the anticarcinogenic effect. ECT is a locally enhanced chemotherapy that combines the administration of a chemotherapeutic drug with cell membrane electroporation (MEP). MEP is the exposure of biological membranes to high external electric pulses, resulting in a destabilized surface tension and an increase in permeability of the cell membrane [58]. The latter is used to transfer into the cytosol large hydrophilic molecules such as bleomycin (BLM) or cisplatin (CIS) that are usually too big to cross the intact cell membrane. Regarding the technical implementation, after intravenous or intratumoral administration of the dedicated anticancer drug electrodes located around or inside the tumor deliver defined electric pulses which enables the cell membrane permeation and consequently the diffusion of otherwise non-permeant drugs into the target cells. Although in ECT the increase in cell membrane permeability is only temporary and reversible, the cytostatic drug once located intracellularly will cause multiple DNA breaks (BLM) or intra- and inter-strand DNA bonds (CIS). MEP in superficial lesions is achieved by the use of electrodes with a fixed geometry, while in deep-seated lesions it is achieved by interaction of single electrodes. Tumor size, location and histological type as well as electrode geometry, number, and spacing determine the effectiveness of ECT. Especially in deep-seated targets parallel long-needle positioning needs the skills and the support of image guidance. The applied electric field needs to be precisely planned to ensure complete and homogeneous coverage of the tumor volume. Dedicated software calculates the optimized placement of the electrodes as well as the voltage for each couple of electrodes. ECT allows controlled locoregional chemotherapy without marked systemic side effects. Moreover, one major benefit of ECT is a low dose of cytostatic drug infused due to a locally increased cytotoxic effect (in vitro 700-fold increase of toxicity in BLM and up to 12-fold in CIS), as only a few molecules per cell are needed to induce cell death [59].
Electrochemotherapy is already well established for cutaneous and subcutaneous primaries and metastases [60]. Preliminary results of the limited publications show that ECT in the liver is also feasible and safe. Data suggest effective local tumor control without compromising the quality of life of patients [61]. In patients with HCC (open surgery) ECT combined with BLM obtained a complete response of 88.2% per lesion [62], in patients with unresectable colorectal liver metastases (CRLM) of 85% [63]. The analysis also revealed that after ECT treatment, most vessels (> 5 mm) and biliary structures were preserved [64]. These findings suggest that ECT can be indicated for the therapy of metastases near major blood vessels in the liver to provide a safe approach with good antitumor efficacy (Figure 3).
Fig. 3
A) Recurrent colorectal liver metastasis at the resection margin after hemihepatectomy. Systemic chemotherapy was exhausted and not well tolerated. B) After intravenous administration of bleomycin electric pulses were deployed through the ECT electrodes. C) 48 hours after intervention contrast enhanced MRI reveals extended destruction of the complete metastasis respecting the tumor margins
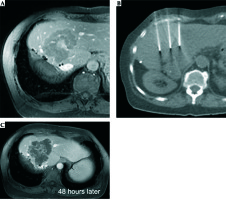
Electrochemotherapy requires general anesthesia due to the electric pulses necessary for MEP. Further, the pulses may interfere with the heart-internal excitation-conduction-system; for that reason ECG-synchronization is mandatory to ensure the pulse delivery in the refractory phase of the heart. BLM may cause lung fibrosis; a higher BLM dosage, older age, and renal insufficiency seem to engrave the risk. BLM has a maximum lifetime dose of 350 mg.
Interstitial interventional radiotherapy (brachytherapy, IRT)
Interstitial interventional radiotherapy (IRT) is also a nonthermal ablation, rather a radioablation. It is an interventional image-guided internal radiation technique for local radiation treatment using high-dose-rate remote afterloading technology. The IRT radiation sources (usually 370 GBq initial activity 192Ir) are temporarily placed via inactive applicators directly into the tissue of concern. The inherent property of IRT is to deliver a very high dose around the source positions and due to the rapid dose fall-off to spare sensitive normal tissues [65].
The optimal placement of the inactive IRT catheters should follow a certain geometry to offer ideal target volume distribution of the potential source positions within the target volume and is usually carried out in the CT scan room under aseptic precautions and under sedation. Once the implantation of the target is completed a thin-slice CT scan is done for dose distribution planning in an adequate treatment planning software package (TPS). The delineation of the clinical target volume (CTV) comprising the gross tumor volume (GTV) and the organs at risk (OARs) is done. After the catheter and anatomy reconstruction in the TPS, a volume optimized dose distribution is created. All modern remote HDR afterloading machines are eligible to deliver the radiation. Typical fraction doses covering the target are 15-20 Gy and usually a single fraction treatment is applied. Due to the steep dose fall-off of the used radioactive source, > 50 Gy in the central tumor region results in the clearance of the central (hypoxic and less radiosensitive) parts of the tumor [66]. The duration of the radiation ranges from 20 to 60 minutes depending on the size of the CTV and the actual activity of the iridium source. The tumor volume can be partitioned to perform sequential treatments if the estimated duration of irradiation exceeds this limit [67]. After treatment is delivered, the brachytherapy catheters are removed.
Computed tomography-guided HDR IRT has generally a very low complication rate. Possible complications may be related to the insertion of the afterloading catheter, but using CT guidance should minimize this. Although IRT lessens radiation damage to healthy tissue due to precise targeting, radiation delivery and protection of radiosensitive organs such as the stomach or intestines are mandatory. To improve the protection of OARs, some groups reported early experience with the successful use of inflatable tissue separation devices [68]. Following IRT in very large tumors, the necrosis may cause temporary fever, chills, and nausea. These side effects may develop about 4-6 hours after the intervention and will disappear after a couple of hours under medication.
Interstitial interventional radiotherapy has some inherent advantages over both thermal ablation techniques and conventional radiotherapy. In contrast to thermal ablations, IRT has no limitations regarding the tumor size that can be treated. Several studies suggest that IRT is also suitable for the treatment of very large liver tumors. Further, the problem of heat dissipation does not occur with IRT. Therefore, high tumor blood flow and nearby larger blood vessels do not affect the success of IRT [67]. In addition, IRT is superior to conventional radiotherapy in terms of the precision of the local radiation delivery into cancer tissue while optimally sparing surrounding healthy tissue [69].
Interstitial interventional radiotherapy was reported to achieve good local tumor control in both primary liver tumors, e.g. hepatocellular carcinoma (HCC) and cholangiocellular carcinoma (CCC), and metastases [66].
In patients with HCC, IRT achieves excellent local tumor control (LTC) of up to 96.1% as well as a high survival benefit compared to the best supportive care (mOS 23 months vs. 5 months) [69]. Furthermore, in secondary liver malignancies, IRT obtains promising local tumor controls of 74.9-97.4%, depending on the primary entity (74.9-87.1% in colorectal cancer (CRC), 96.5-97.4% in breast cancer and 90% in pancreatic cancer liver metastases) [69,70,71,72,73] (Figure 4).
Radioembolization
Selective internal radiotherapy (SIRT) is the endovascular approach of delivering focal radiation that employs radioactive isotopes, e.g. yttrium-90 (90Y)-tagged glass or resin microspheres. SIRT is technically a whole-liver therapy. Tumor selectivity is achieved by the predominant supply of hypervascular malignancies by the hepatic artery [74]. After injection into the right or left branch of the hepatic artery, the microspheres become preferentially lodged in the arteriolar vasculature surrounding the tumor, delivering high doses of radiation to the surrounding area. Maximum tissue penetration for the pure beta-emitter 90Y is 1.1 cm. Absolute contraindications for SIRT are a potential for ≥ 30 Gy radiation to be shunted to the lungs attested in the pretreatment technetium-99 (99Tc) scan, inadvertent flow to the gastrointestinal tract that cannot be corrected with protective embolization, and prior radiation therapies of the liver. Unlike transarterial chemoembolization (TACE), main portal vein thrombus or obstruction is not a contraindication to radioembolization. Arterial ischemia after radioembolization based on the size of the beads is negligible, so the portal blood supply is less important from the standpoint of toxicity. In HCC SIRT induces extensive tumor necrosis with an acceptable safety profile [75]. However, there is no consensus as to the optimal use of this therapy, particularly when and if it should be chosen over TACE for treatment of unresectable HCC [76,77,78,79]. For patients who are eligible for TACE, generally TACE is still suggested. SIRT is preferred over TACE for patients with advanced HCC who are otherwise adequate candidates for local embolization therapy but who have macrovascular invasion, such as portal vein thrombus [80]. In symptomatic patients with metastatic carcinoid and pancreatic NET SIRT is also an alternative palliative technique to medical therapy alone [81,82]. In metastatic CRC randomized trials suggest that SIRT combined with systemic therapy does not improve survival as compared with chemotherapy alone and is associated with more adverse events. In the combined analysis of all three trials (SIRFLOX, FOXFIRE, and FOXFIRE-Global, 1103 patients, 549 receiving chemotherapy alone and 554 receiving chemotherapy plus SIRT), at a median follow-up of 43 months, despite having a higher objective response rate with combined therapy (72% vs. 63%), this did not translate into improved median overall survival (22.6 vs. 23.3 months, hazard ratio [HR] 1.04, 95% CI: 0.9-1.19) or progression-free survival (11 vs. 10.3 months, HR 0.90, 95% CI: 0.79-1.02), or a greater likelihood of subsequent liver resection. Furthermore, combined treatment was associated with significantly more grade 3 or 4 adverse events (especially hematologic toxicity). Of the 11 treatment-related deaths on study, eight were in the chemotherapy plus SIRT group, three of which were attributed to radiation-induced liver disease [83,84,85,86]. Further studies have to define the role of SIRT as consolidation therapy after chemotherapy.
Economic aspects
The cost evaluation of different treatment methods is very complicated and the result depends on many variables [87]. Halpern et al. performed a comparative cost analysis in the USA [88], which resulted in median cost with SBRT in $27,145 compared to $17,183 for brachytherapy, $37,090 for IMRT and $54,706 for proton beam therapy (p < 0.001).
We also present the actual reimbursement costs for the different local ablation methods of percutaneous liver tumor ablations in Germany (Table 1).
Table 1
Reimbursement of different non-surgical percutaneous tissue ablation methods in Germany (2019)
Status of education in interventional oncology
Patients with cancer deserve evidence-based care, including the delivery of high-quality, high-value local treatments. Continued education is mandatory to advance awareness of and expertise in the full spectrum of interventional oncology applications [89,90]. In the interventional radiology community the need of education and quality assurance was realized [91,92] and CIRSE (Cardiovascular and Interventional Radiology Society of Europe) published recommendations for IR. Although basic knowledge of stereotactic and interventional radiotherapy is included in the published “European Curriculum and Syllabus for Interventional Oncology”, no detailed knowledge of this method is disseminated in the organ-specific chapters [93].
In radiotherapy, ESTRO (European Society for Radiotherapy and Oncology) and GEC (Groupe Européen de Curiethérapie) offer multidisciplinary teaching courses and organ-specific treatment recommendations, although IO specific activities are rare. However, at the national society level, there is a growing number of activities to improve the situation [94,95,96,97].
Discussion
Thermal ablation techniques are emerging as alternative treatment options to open surgery, being a well-recognized treatment option for both primary and secondary hepatic tumors [22]. However, hyperthermia-based technologies have some limitations, including heat sink effects in the vicinity of large blood vessels, the risk of causing cholestasis when treating lesions close to the thermosensitive bile ducts or damaging critical structures if proximal to the hepatic portal Glisson’s capsule or diaphragm, or if located on the intra-abdominal free surface [98]. Since the liver is an organ of particular interest in interventional oncology, non-thermal ablation (NTA) techniques have the potential to complement local tumor therapy beyond the limitations of already established thermal ablations such as RFA or MWA. The lack of thermonecrosis and the preservation of collagenous structures enables NTA to be used near sensitive structures such as vessels, and bile ducts and may open up new opportunities for minimally invasive treatments in selected settings, e.g. large liver tumors located at the hilum of the liver.
Electrochemotherapy and IRT both have resemblances with endovascular interventional oncologic therapies. Similar to TACE, ECT enables controlled locoregional chemotherapy without marked systemic side effects. Using MEP, ECT has the potential to achieve more homogeneous concentrations of a cytostatic drug in big lesions, assuming that response rates achieved with ECT may exceed those achieved with TACE [99]. Analogous IRT resembles selective internal radiation therapy (SIRT), allowing a welldefined focal to regional high-dose radiation preserving the functional reserve of the healthy liver remnant. In other anatomic sites, IRT was also proven as a successful treatment in the elderly and frail population [100,101].
This potential advantage of HDR-IRT seems to be more important if we have a look at the recent publication of Gomez et al. reporting local treatments in patients with oligometastatic non-small cell lung cancer that did not progress after front-line systemic therapy, with prolonged progressionfree survival and overall survival obtained compared to maintenance therapy or observation. Even if the randomized phase II study of Palma et al. showed a significant increase in terms of overall survival for oligometastatic disease with stereotactic ablative radiotherapy (SABR), the treatment was associated with death related to the procedure in 4.5% of patients (3/66). Toxicities causing deaths were related to radiotherapy (radiation pneumonitis, pulmonary abscess and subdural hemorrhage after surgery to repair a SABR-related perforated gastric ulcer) [14]. Comparable toxicities are not reported in the HDR IRT literature.
Conclusions
Despite the growing evidence for practicing hypo-fractionated regimes in many solid malignancies as well as the inherent benefit of delivering a high dose with a rapid dose fall-of, the limited use of IRT in comparison to conformal (3D-EBRT) or stereotactic external beam (SBRT) radiotherapy may be due to its invasive approach, operative risk, technical challenge, and long learning curve. Multicenter databases are mandatory for providing scientific evidence for eventually increasing the use of IRT in clinical practice, including for liver lesions.
Because of the need of the experience of different specialties, successful IRT is usually performed in a multidisciplinary cooperation by interventional radiologists and interventional radiation therapy experts. In such multidisciplinary groups, the potential of advising/applying the best fitting personalized local ablation method is also mandatory.