Maintenance of adequate urine flow during surgical procedures is a major concern for the anaes-thetist. In such a context, oliguria can occur if the kidneys are poorly perfused or the arterial pressure is low, which increases the risk of postoperative acute kidney injury (AKI) [1, 2]. Oliguria may also be caused by hormonal factors and by general anaesthesia or possibly by certain anaesthesia drugs. Specifically, volatile anaesthetics have been suspected of having an intrinsic depressive effect on urine output. Therefore, the role of anaesthetic drugs in the development of oliguria during and after surgery should be clarified.
Isoflurane was found to have a depressive effect on urine output in sheep 20 years ago [3, 4] and was also associated with a greater decrease of the cortical and medullary blood flow rates than propofol [5]. A study in humans showed that urine output was lower and the incidence of postoperative AKI higher in patients anaesthetized with sevoflurane rather than propofol [6]. Franzén et al. [7] recently reported lower urine output in patients undergoing spinal surgery using sevoflurane compared to those given propofol. Volatile anaesthetics may also exert a toxic effect on the kidneys via increased plasma fluoride and compound A concentrations [8] but the risk of such complication seems to be small with normal use [9, 10].
Urine production is a multifactorial process, and the diuretic response to a fluid load, which is commonly used to study this topic, is dependent on the degree of fluid-induced plasma volume expansion, the choice of fluid, the infusion time and observation time, plasma creatinine, arterial pressure, and hormonal responses [11–14]. How the kidneys are pre-set to excrete or conserve water also plays an important role in urine output [14, 15].
The present study re-evaluates the proposed difference in diuretic response between volatile and intravenous anaesthesia by performing volume kinetic analysis of crystalloid fluid infused during ongoing surgery. This method captures renal efficiency in excreting fluid by calculating a rate constant k10, which is the measured urine output divided by the plasma volume expansion over time [16]. This approach more accurately quantifies the “diu-retic response” than urine output alone, as k10 is corrected for plasma volume expansion and for variations in observation time. Moreover, if statistically justified, k10 can also be corrected for confounders such as arterial pressure, age, and sex.
The evaluation used data from two clinical trials where surgical patients were randomised to receive isoflurane or propofol [17] and sevoflurane or propofol [18]. The hypothesis of the study was that renal efficiency is decreased by general anaesthesia per se and not by a specific effect of volatile anaesthetics.
Methods
Patients
Material for the present analysis was derived from a database with intravenous infusion experiments. Data sets that represented two complete clinical trials were chosen, where a total of 56 elective patients undergoing open thyroid surgery [17] or open hysterectomy [18] were randomised with the sealed envelope technique to receive general anaesthesia using isoflurane/sevoflurane or propofol augmented by intermittent boluses of fentanyl.
The studies were approved by the Regional Ethics Committee of Huddinge Hospital (269/02) on September 2, 2002, and by the Ethics Committee of Riga Stradins University on January 27, 2016. The latter study was registered at controlled-trials.com as ISRCTN81005631.
All patients provided written informed consent to participate. Inclusion criteria were ASA I–II, age 18–90 years and scheduled elective thyroid surgery or open hysterectomy. Exclusion criteria were cardiopulmonary or renal disease requiring daily the-rapy, cancerous diagnosis, plasma creatinine above the normal range, expected operating time < 90 min and blood loss > 500 mL, emergency surgery, pregnancy, regional anaesthesia, and ASA class III–IV. Reporting followed the TREND statement checklist.
Procedure
Patients fasted overnight and received no premedication (including diazepam) by mouth. The ope-rations started between 8:00 and 9:00 a.m. at Södersjukhuset in Stockholm, Sweden, and at Paul Stradins Clinical University Hospital in Riga, Latvia. General anaesthesia was induced with propofol and fentanyl, after which tracheal intubation was facilitated with atracurium. In one group, anaesthesia was maintained with continuous administration of propofol augmented with intermittent injections of fentanyl. In the other groups, anaesthesia was maintained with isoflurane (thyroid study) or sevoflurane (hyste-rectomy study). Pulmonary ventilation was based on low-flow anaesthesia where the tidal volume was adjusted to achieve normocapnia. Patients undergoing thyroid surgery were placed in the horizontal recumbent position and the hysterectomy patients either in the horizontal position or 5% Trendelenburg position. Monitoring consisted of electrocardiography, pulse oximetry, and non-invasive heart rate and arterial pressures (D-LCC15.03 or AS-3, Datex-Ohmeda, Finland). No fluid was administered during onset of the anaesthesia. Twelve patients in the isoflurane group of the thyroid study were given an intravenous injection of 5 mg of ephedrine, which could be repeated if their mean arterial pressure decreased below 55 mmHg during the induction. No hysterectomy patient was given a vasopressor. One patient in the propofol group in each trial was excluded due to major haemorrhage (> 1 L).
Fluid programmes and blood sampling
All patients received 25 mL kg–1 of Ringer’s ace-tate [17] or Ringer’s lactate [18] intravenously over 30 min, beginning when surgery had just started. In the thyroid study, blood samples (3 mL) were withdrawn for measurement of the venous haemoglobin (Hb) and plasma albumin concentrations immediately before the infusion of fluid was initiated (duplicate), every 5 min during the infusion, for 30 min thereafter, and at 15–30 min intervals for a total length of up to 150 min. In the hysterectomy study, blood was sampled every 10 min up to 90–100 min. Hb and plasma albumin were analysed in the hospital’s certified clinical chemistry laboratory with coefficients of variation of approximately 1% and 2%, respectively. A urinary catheter was placed in the bladder after anaesthesia had just been induced. Urine output was measured every 30 min (3–5 times during each operation).
Kinetic analysis
The population (mixed models) method used in the present study is a standard industry procedure for analysing the kinetics of drugs that is based on likelihood mathematics. The model consisted of three functional fluid compartments and was fitted to the frequent measurements of Hb-derived plasma dilution and the urine output. The first step was to estimate the basic (fixed) parameters that governed the fluid distribution, which comprised five rate constants (k12, k21, k23, k32, and k10) and one calibration factor between plasma dilution and plasma volume (Vc) (Figure 1). The second step was to include covariates. These are individual-specific or group-specific factors (sex, age, arterial pressure, etc.) that make the model predictions more precise in each patient. A covariate can also be used to statistically evaluate differences between subgroups (such as various choices of anaesthesia) when all other variables in the model are considered.
FIGURE 1
Schematic drawing of the kinetic model used to analyse the distribution of Ringer’s solution. Special focus was on obtaining an accurate estimate of k10
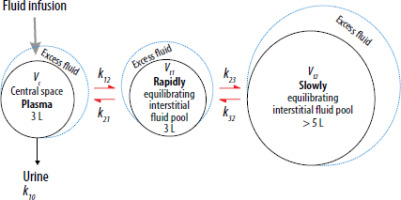
The most important model parameter in the present study was k10, which is the rate constant describing the diuretic response to fluid. This rate constant equals the ratio between the measured urine output and the area under the curve for the volume expansion of the central space (Vc, the functional plasma volume) [16].
The three-compartment model was simultaneously fitted to all measurements of plasma dilution and to urinary excretion (dependent variables) in each of the two studies, using the Phoenix software for nonlinear mixed effects (NLME), version 1.3 (Pharsight, St. Louis, MO) with the First-Order Conditional Estimation Extended Least Squares (FOCE ELS) as a search routine. The Supplementary File contains details about calculations, differential equations, search strategies to find covariates to the fixed parameters, and how they should be interpreted.
The isoflurane study has previously been analysed using the two-step method, which means that a separate analysis was made for each patient. Therefore, a new analysis was performed by using a population volume kinetic approach guided by recent advances in the organization of the interstitial fluid space [19, 20].
The sevoflurane study has recently been subjected to population kinetic analysis [18] and did not undergo the same analysis again. However, the present study tested the add-on of a potential difference in k10 depending on the use of sevoflurane instead of propofol.
Statistical analysis
Data showing a normal distribution are reported as the mean and standard deviation (SD) and compared with one-way analysis of variance (ANOVA). Data with skewed distributions are given as the median (25th–75th percentiles limits) and differences between subgroups were analysed using the Mann-Whitney U test. Statistical analysis was performed using SPSS 28.0.1.1 (IBM, Armonk, NY). P < 0.05 was considered statistically significant.
Kinetic parameters are reported as the best estimate (95% confidence interval) according to the output from the Phoenix program. The significance levels for inclusion of the covariates were taken from the Phoenix program. The difference between intravenous and volatile anaesthesia was evaluated statistically by the Phoenix program based on whether inclusion of volatile anaesthesia reduced the –2 log likelihood for the model by > 3.8 (P < 0.05). A χ2 P-value is given based on the likelihood ratio test.
A post hoc power analysis based on k10 of the thyroid study, where the best estimate was 2.45 × 10–3 min–1 and the standard error of 0.36 × 10–3 min–1, reached a power of 94% to detect a difference of 20% at the significance level of P < 0.05. The hysterectomy study had a best estimate of k10 of 0.86 × 10–3 min–1 and standard error of 0.072 × 10–3 min–1, which yielded a power of 96% to detect a difference of 20% at the significance level of P < 0.01.
Results
Urine output
Age and body weight did not differ between the subgroups that received inhaled and intravenous anaesthesia (Table 1). All patients received 25 mL kg–1 of fluid over 30 min.
TABLE 1
Demographic and perioperative data in two studies where patients had been randomised to receive volatile anaesthesia or a propofol infusion supplemented with intermittent fentanyl
The median urine output for propofol vs. inhaled ana-esthesia was 132 (77–231) mL vs. 218 (80–394) mL at 150 min in the isoflurane study (P = 0.50) and 50 (45–65) vs. 60 (34–71) mL at 90 min in the sevoflurane study (P = 0.81; Figure 2).
Kinetics in the isoflurane study
The measured plasma dilution (631 data points) and urine output (138 data points) were compared to the corresponding values predicted by the kinetic model in Figures 3A and B. The confidence limits for predicted and measured plasma dilution values are shown in Figure 3C.
FIGURE 3
Kinetic analysis of 25 mL kg–1 of Ringer’s acetate given over 30 min to patients undergoing thyroid surgery. A) Comparison of measured plasma dilution and the individual values predicted by the kinetic model. B) Same comparison but for urine output. C) Confidence limits for the plasma dilution as given by the observed data and the same limits when these data were recreated by 1,000 simulations using the best estimates of the kinetic parameters. D) Covariate analysis of the relationship between the elimination rate constant k10 and the systolic arterial pressure. E) Covariate analysis of the relationship between k10 and patient age. F) Simulation of the distribution 1.7 L of Ringer’s acetate infused over 30 min between plasma and two interstitial fluid compartments. All plots were derived by the Phoenix NLME program
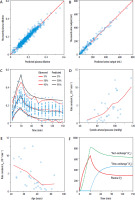
The final kinetic parameters are shown in Table S1 of the Supplementary File. The search for covariates identified a positive correlation between the systolic arterial pressure and the rate parameter for urine flow (k10, Figure 3D). Age correlated inversely with k10 (Figure 3E). Among patients administered ephe-drine there tended to be a higher value of k12, which is the rate constant governing the capillary leakage rate, but the covariance was not statistically significant. The model-predicted distribution of the infused fluid between the body fluid compartments is shown in Figure 3F.
The choice of isoflurane instead of propofol had no statistically significant influence on k10. This was shown by the fact that –2 log likelihood (–2LL) for the kinetic model only decreased from –1330.3 to –1330.4 when isoflurane/propofol was introduced as a potential covariate to k10 (P = 0.80; Figure 4A), whereas a decrease in –2LL by 3.8 points is needed to be significant at P < 0.05, or by 6.6 points to be significant at P < 0.01.
FIGURE 4
The distribution of the rate parameter for urine output (k10) in studies where surgical patients were randomised to receive propofol or volatile anaesthetics. A) Kinetic analysis of Ringer’s acetate based on Hb-derived plasma dilution during thyroid surgery. B) Same study, but plasma albumin was used to calculate plasma dilution. C) Kinetic analysis of Ringer’s lactate solution based on Hb-derived plasma dilution during open hysterectomy
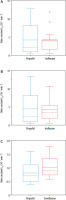
When albumin was used to calculate the plasma dilution, the addition of isoflurane/propofol to the final model decreased –2LL from –949.6 to –949.7 (Figure 4B).
Kinetics in the sevoflurane study
The sevoflurane study comprised 299 data points of plasma dilution and 73 of urine output. The –2LL value of the final model based on Hb decreased from –281.1 to –282.1 when sevoflurane/propofol was tested as a covariate to k10 (P = 0.12; Figure 4C).
When using albumin to calculate dilution, the addition of sevoflurane/propofol as a covariate only decreased –2 LL from –263.4 to –264.7 (P = 0.25; data not shown).
Discussion
Key findings
The present analysis does not support the notion that volatile anaesthetics depress urine output more than intravenous anaesthesia using propofol/fentanyl does. This conclusion is based on the analysis of two randomized clinical trials where the urine output following volume loading with Ringer’s solution was quite similar regardless of type of anaesthesia. More importantly, volume kinetic ana-lysis based on serial analysis of both blood Hb and plasma albumin did not show that the use of volatile anaesthetics served as a covariate to the elimination rate constant (k10). The log likelihood output from the kinetic analysis did not even provide weak evidence of a specific effect of isoflurane or sevoflurane on k10. This parameter provides a more accurate quantification of “diuretic response” to fluid than urine output alone, as k10 is free from several confounders that may affect diu-retic response, including variations in follow-up time and fluid-induced plasma volume expansion. Technically, k10 yields the urine flow rate per minute when multiplied with the plasma volume expansion in mL at any time.
Why urine output is low?
There are several factors that depress urine output during surgery. General anaesthesia decreases the driving pressure for the circulation, the mean circulatory filling pressure, by about 25% [26], which decreases the global perfusion pressure. Low syste-mic arterial pressure unloads the baroreceptors, which increases the activity in the renal sympathetic nerves and, in turn, increases renin secretion and activates sodium and water reabsorption [22, 23]. Taavo et al. [24] recently demonstrated that sevoflurane-induced oliguria in sheep is abolished by denervation of the renal sympathetic nerves, but they attri-buted the oliguria to sevoflurane and not to the arterial pressure. Vasopressin is released in response to visceral traction, which might explain why the open hysterectomies had a lower overall urine flow than the thyroid resections [25]. Elevated plasma creatinine prolongs the elimination of crystalloid fluid, at least in the awake state [12]. Patients with renal water conservation due to low habitual intake of water have a poorer diuretic response to fluid loading in the awake state [14] and during surgery [15]. Cortisol and aldosterone are released due to trauma and poor renal perfusion, respectively, but steroid hormones have a slow onset of action and may be more important during the postoperative period.
Arterial pressure
In awake humans, k10 is approximately 15–25 min–1 [26], i.e., 10 times higher than in the present work. Previous evaluations confirm that general anaesthesia is associated with a depression of the diuretic response to fluid that amounts to 85–90% [14, 20]. In a study of both conscious and anaesthetized humans, this decrease was attributed to low mean arterial pressure and old age [12]. The mean arterial pressure has also been statistically associated with k10 in awake subjects [26] and during induction of general anaesthesia [27], but this covariance has never been challenged in already anaesthetized patients.
In the present study, variations in arterial pressure were small, since all patients were anaesthetized. The arterial pressure correlated with k10 in the thyroid study, although the systolic pressure showed stronger covariance with k10 than the mean arterial pressure did. Therefore, the systolic arterial pressure was selected for inclusion in the kinetic model (Supplementary Table 1). This correlation was also positive in the hysterectomy study, but it did not quite reach statistical significance. However, the urine output was extremely low and vasopressin release or random factors may then have been important.
The value of k10 may also vary in individual patients depending on other covariate effects, such as age, sex, and body weight. In the present study, confounding effects were mostly managed by the highly standardized protocols and the randomization procedure, although their overall influence on urine output was probably limited due to the strong depression of k10 exerted by general anaesthesia per se. The only challenged covariate effect was the influence of volatile anaesthetics on k10.
Acute kidney injury
The urine flow during the surgery was often below the threshold for oliguria at 0.5 mL kg–1 h–1 (Table 1), which is a criterion for AKI if maintained for 6 h according to the KDIGO (Kidney Disease Improving Global Outcomes) criteria [1]. The specific incidences were 24% and 42% in the two cohorts, respectively, while the better predictive threshold for outcome of 0.3 mL kg–1 h–1 [2] was reached in 14% and 15% of the patients. The diuretic response to fluid loading usually becomes normalized soon after anaesthesia ends [28]. However, intraoperative oliguria might still predispose to postoperative AKI when indicated by a rise in plasma creatinine by 50% above the preoperative concentration [2]. A recent study suggested that the combination of low habitual water consumption and intraoperative oliguria increases postoperative plasma crea-tinine sufficiently to be diagnosed as AKI even in the absence of structural or physiological damage to the kidneys [15]. Hence, oliguria is a contributing factor to postoperative AKI but by itself is probably not sufficient to cause this complication.
Evaluating urine output
The difference in urine output between volatile and intravenous anaesthesia in previous research has been statistically significant but still numerically small. Based on 4,320 surgical patients, Bang et al. [6] found that sevoflurane decreased urine output more than propofol, the median values being 240 and 210 mL, respectively. The difference was of similar magnitude in Franzén’s report [7]. However, crude urine output may offer an uncertain basis for judgments about a drug-specific effect in complex clinical settings where the infused volumes are not standardized, continuous infusions of vasopressors are given, follow-up times may differ, blood transfusions are given, and arterial pressure is not closely controlled. The two clinical trials presented here were well standardized regarding surgical procedure, infused fluid volume, fluid type, and infusion time. No fluid was given in addition to the 30-min infusion of crystalloid, and no blood was transfused. The same research team managed all operations in each trial.
Kinetic model
Population kinetics is a standard method used in the study of drugs, but the present approach (volume kinetics) uses measurements of plasma dilution instead of drug concentrations as a dependent variable [16]. Volume kinetics is a whole-body model that identifies time delays in the distribution of fluid from the plasma (Vc) to two peripheral spaces. These delays are quantified by the rate constants k12 and k23 [19, 20]. Fluid passes relatively quickly from the plasma to a “fast-exchange” extravascular space, while transfer to the “slow-exchange” compartment apparently requires that the hydrostatic pressure is increased in the “fast-exchange” compartment (Figure 3F).
Limitations
Limitations include the fact that the analyses were based on retrospective data and that the origi-nal presentations did not focus on urine output. The kinetic analyses were updated to adequately compare volatile to intravenous anaesthesia and their possible relationship with urine output. The arterial pressure showed limited variation but still offered valid information for kinetic modelling by serving as a “time-varying covariate”. This means that the association between arterial pressure and urine output was considered whenever measured and not only based on the mean values for entire expe-riments. However, the covariance plot in Figure 3D summarizes the systolic pressure for one experiment at each point.
The kinetic analyses do not consider the flow rates at baseline. Body fluid constantly circulates from the plasma to the interstitium and back to the plasma via the lymph even if no volume changes occur. On the other hand, urine is also produced even if no plasma volume expansion occurs, which then induces dehydration and hypovolaemia. A baseline diuresis of approximately 1 mL per min could be considered in the analysis, but it still had to be matched by infused fluid to achieve a positive plasma dilution. Therefore, making such a correction can be questioned. However, a complete view of the hormonal responses to fluid loading was not included in the kinetic analysis.
Ringer’s acetate was used in the thyroid study, while the hysterectomy patients received Ringer’s lactate. A previous analysis showed that the choice of Ringer solution has a negligible influence on the fluid kinetics [12].
Conclusions
The present evaluation does not support the notion that volatile anaesthetics need to be avoided to prevent intraoperative oliguria. In two randomized clinical trials, urine output and the kinetic rate constant representing the diuretic response to volume loading did not differ between patients anesthetized with volatile anaesthetics (isoflurane or sevoflurane) and intravenous anaesthesia. Oliguria is more likely to be dependent on the depth of the anaesthesia and other factors than on the type of anaesthesia. This result is relevant because previous studies have reported that a statistically significant, but still not very strong, relationship exists between intraoperative oliguria and postoperative AKI.