Purpose
Cervical cancer is a common cause of death among women worldwide, and about 230,000 women die of cervical cancer every year in developing countries [1]. Surgery, radiation therapy, and concurrent chemoradiotherapy are the main treatment options for cervical cancer. As an essential adjuvant radiation therapy, brachytherapy plays a crucial role in the management of invasive cervical cancer, and can significantly improve local control rate of tumor and patients total survival rate [2-5]. With the development of three-dimensional (3D) imaging techniques, such as computed tomography (CT), magnetic resonance imaging (MRI), and positron emission tomography (PET)-CT, image-guided brachytherapy (IGBT) have become widely used, which enabled 3D brachytherapy to significantly reduce the dose to organs at risks (OARs) while ensuring satisfactory target coverage [6-9]. According to the tumor location, size, and complexity in different patients, several applicators exist for high-dose-rate (HDR) brachytherapy. For locally advanced cervical cancer, brachytherapy methods mainly include intra-cavity (ICBT), interstitial interpolation (ISBT), or intra-cavity combined with interstitial interpolation (IC + ISBT). However, there is no consensus regarding the optimal applicator delivery selection method [6, 10, 11].
For ICBT patients treated using a fixed applicator, i.e., the Elekta’s titanium Rotterdam three-tube applicator, an MR-compatible metal applicator has its unique advantages [12]. The applicator can save operation time, improve efficiency of the procedure, is less risky for patients, and cost-effective. However, not all patients are suitable for ICBT. For some cases with complex tumors, IC + ISBT, a 3D printing technique, may be more suitable because of the limitation regarding the standard size for intra-cavity fixed applicator technique [13-15].
Previous studies performed comparative analysis between IC + ISBT and ICBT alone, but the target volume was rarely taken into consideration. Therefore, for a certain tumor volume, to ensure the protection of OARs and target coverage, the choice of applicator is very important. Since each applicator has its own specific shape of dose distribution, to obtain the best treatment outcomes, the applicator should be selected according to the target shape and size [16-18].
In this study, target volume was taken into account, and plans were categorized into different categories based on target volume. For each target volume category, 3D-printed and Rotterdam three-tube (TT) groups were compared. We conducted a retrospective study to investigate the dosimetric and plan parameter differences between IC + ISBT, involving the combination of uterine tube and implantation needles for 3D-printed templates and CT-guided ICBT with fixed TT applicator, to provide meaningful reference for clinical practice in HDR brachytherapy of cervical cancer.
In this study, D2cc of OARs, D90 and D98 target coverage, and plan parameters were compared between 3D-printed and TT groups. The purpose of this study was to investigate the clinical outcomes and dosimetric parameters of patients treated with IC + ISBT according to different target volume categories. We sought to determine a patient target volume threshold most suitable for 3D printing technique. Therefore, the aim of the present study was to explore the feasibility of 3D-printed technique in IC + ISBT, and provide a clinical reference for HDR treatment in locally advanced cervical cancer.
Material and methods
Patient population
A retrospective study of 100 patients with locally advanced cervical cancer treated with CT-guided three-dimensional brachytherapy, admitted to our hospital from May 2019 to May 2022. According to treatment methods, patients were divided into IC + ISBT and ICBT groups. This retrospective study included a total of 400 fractions plans for 100 patients. The selection of applicator was determined according to tumor stage, tumor size, tumor invasion mode, equivalent biological dose, and whether the patient received chemotherapy or not before treatment. Characteristics of the patients (age range, 32-76 years; median, 52.9 years) are presented in Table 1.
Table 1
Characteristics of the patients
FIGO stage | Total number (%) | Synchronous chemotherapy | Age | ||
---|---|---|---|---|---|
Yes (number) | No (number) | Range (years) | Median (years) | ||
IIB | 30 | 94 | 6 | 32-76 | 53 |
IIIB | 22 | ||||
IIIC1 | 26 | ||||
IIIC2 | 10 | ||||
IVB | 12 |
Each patient received external radiation dose of 56 Gy in 28 fractions, or 50.4 Gy in 28 fractions. The total prescribed brachytherapy dose for each patient was 26 Gy in 4 fractions (6.5 Gy per fraction), administered once a week.
Process Of Operation, pre-planning, and treatment
For all patients who underwent conventional surgical procedures, a pre-operative indwelling catheter was placed, and disinfection was done by placing towels under patients bodies. Patients were encouraged to empty their bowels, and 100 ml of normal saline was injected. All patients were operated on a transfer bed in a lithotomy position. After the surgery, appropriate anterior and posterior vaginal packings were applied to fix the applicator into position and displace OARs away from the applicators. A consecutive CT scan with 3 mm slice was performed. Then, HR-CTV and OARs were delineated by gynecologic oncologists on CT images according to the Groupe Européen de Curiethérapie and European Society for Radiotherapy and Oncology guidelines [19]. Treatment plans were done using Oncentra (Nucletron, Elekta) treatment planning system. Figure 1 shows 3D-printed and TT applicators, and an example of dose distribution of different plans according to two-applicator treatment modes under CT guiding.
Fig. 1
Images of the two treatment group applicators and dose distribution for one patient in each group. A) 3D-printed template applicator. B) Dose distribution in the tomographic plane of a patient in the 3D-printed group. C) Dose distribution in the coronal plane for the same patient as in (B). D) Dose distribution in the sagittal plane for the same patient as in (B). E) Rotterdam three-tube applicator. F) Dose distribution in the tomographic plane of a patient in the Rotterdam three-tube group. G) Dose distribution in the coronal plane for the same patient as in (F). H) Dose distribution in the sagittal plane for the same patient as in (F). Red dotted line represents the delineation of the target HR-CTV, while yellow solid line shows the area surrounded by 100% of the prescribed dose
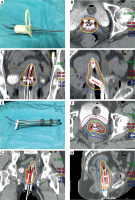
For patients in the 3D-printed group, pre-planning occurred first using the following rules: 1) The total dose is the superposition of the dose of external radiation and brachytherapy. According to a linear quadratic equation, it is equivalent to the conventional split dose of 2 Gy (EQD2). The α/β ratio of tumor is 10, and that of OARs is 3. The dose of HR-CTV D90 is ≥ 85 Gy, for the bladder is ≤ 90 Gy, and for the rectum, sigmoid, and bowel it is ≤ 75 Gy; 2) The needles should meet clinical practicability, such as the minimum and maximum spacing distance between the needles; needle channels should not cross and be on the template, and they cannot be inserted into patient OARs; 3) For the depth of the needle inserted into the tissue, the offset length at the front end of the needle should be included; 4) Template-personalized printing include channel position, channel angle, and channel length for each parallel and oblique needles.
Data collection of dosimetric and plan parameters
In our study, data of 100 patients (50/50, treated with 3D-printed/TT applicators) were used for brachytherapy, and HR-CTV distribution was determined for 400 plans; the target volume distribution of the two groups conformed to normal distribution and were comparable in quantity (Figure 2). From these 400 fractions plans, we categorized 377 plans into six volume categories at intervals of 10 cm3 according to distribution of HR-CTV.
Fig. 2
Histogram of the distribution between the HR-CTV volume size and the number of plans between the 3D-printed and Rotterdam three-tube groups, A) the 3D-printed group and B) the Rotterdam three-tube group.
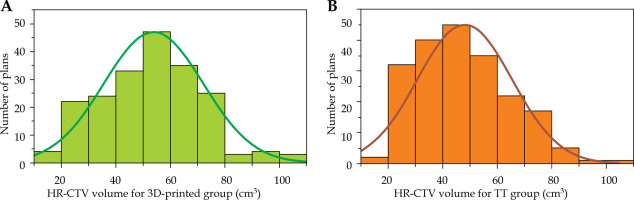
To obtain more reliable results, the size of target volumes for each of six volume categories in both 3D-printed and TT applicator groups were compared. Then, the dosimetry and planning parameters in these six volume categories for the two groups were compared, so that the target volume threshold category that was the most suitable for 3D printing therapy could be determined.
Data of HR-CTV D90, HR-CTV D98, and D2cc of the bladder, rectum, sigmoid, and bowel were collected. Homogeneity index (HI), conformation number (CN), and sparing factors (SFs) of each OARs were calculated based on the data above. HI, CN, and SFs were calculated using formula reported by Ren et al. and Oku et al. [20, 21]. HI referred to the ratio of difference between the planned volume irradiated at 100% and that at 150% of the prescribed dose to the volume irradiated at 100% of the prescribed dose, which represented the uniformity of the plan. CN was calculated as the multiplication of two ratios (ratio of the volume irradiated at 100% of the prescribed dose to the target of HR-CTV multiplied by the ratio of the volume irradiated at 100% of the prescribed dose to the volume of HR-CTV receiving great than or equal to 100% of the prescribed dose area). The closer the CN value was to 1, the higher the conformity of the target. SFs of OARs referred to the ratio of D2cc corresponding to OARs of HR-CTV D90. The smaller the SF, the better the protection of OARs.
Statistical analyses
According to the two applicator treatment modes, 377 plans were compared and analyzed using IBM SPSS Statistics for Windows, version 27.0 (IBM Corp., Armonk, NY, USA). Mann-Whitney U test was performed to compare the six categories of the plans between the two groups. Dosimetry parameters (HR-CTV D90, HR-CTV D98, and D2cc of OARs) and planning parameters (CN, HI, and SFs of OARs) were all collected, analyzed, and compared for each of the six HR-CTV volume plan categories between the 3D-printed and TT groups. P-value ≤ 0.05 was considered statistically different.
Results
Table 2 shows the comparison results between 3D-printed and TT applicators groups under different HR-CTV categories. Figure 2 presents the distribution between the number of plans and the size of target volume for each of the two groups. The number of plans for the six volume categories were comparable between the two groups. There were no significant differences in all the six target volume categories between the two groups (showed in Table 2). Therefore, we investigated whether the effect of these two treatment techniques was related to the volume of the target.
Table 2
Comparisons in statistical dosimetric and planning parameters between the two applicator groups
[i] 3D – 3D-printed template-based applicator group, TT – Rotterdam three-tube fixed applicator group, HR-CTV – high-risk clinical target volume, HR-CTV D90 – the dose that covers 90% of the target volume, HR-CTV D98 – the dose that covers 98% of the target volume, D2cc – the minimum dose in the most irradiated 2 cm3, SF – sparing factor, the smaller the value: the lower the exposure dose to organs at risk, HI – homogeneity index: the closer the value to 1, the better the uniformity, CN – conformity index: the closer the value to 1, the better the conformity
Compared with the TT group, when the HR-CTV category ranged from 20-30, 30-40, 40-50, 60-70 to 70-80 cm3, the average of dosimetry parameters, including D90 and D98 of HR-CTV in the 3D-printed group were generally higher than that for the TT group; however, the difference was not always significant. Whereas, the 3D-printed group D2cc of OARs (the bladder, rectum, sigmoid, and bowel) were lower overall compared with the TT group, although the difference was not always significant. Moreover, SF planning parameter showed similar results.
When the HR-CTV category ranged between 50-60 cm3 compared with the TT group, the average D90 and D98 of HR-CTV in the 3D-printed group was significantly higher (p < 0.05, Figure 3), whereas the mean D2cc of OARs were significantly lower (p < 0.05, Figure 4). The SFs for each OAR, HI, and CN were better than those in the TT group (p < 0.05, Figures 5 and 6, respectively).
Fig. 3
Box plots of the comparison results of D90 and D98 HR-CTV for the plans of volume size of HR-CTV of 50-60 cm3 between the 3D-printed and Rotterdam threetube groups
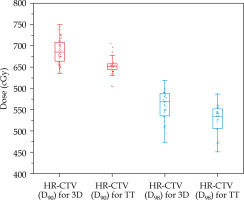
Fig. 4
Box plots of the comparison results of D2cc of the bladder, rectum, sigmoid, and bowel for the plans of HRCTV volume size of 50-60 cm3 between the 3D-printed and Rotterdam three-tube groups
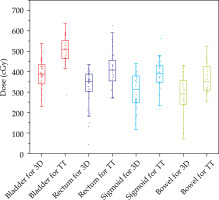
Fig. 5
Box plots of the comparison results of planning parameters sparing factors (SFs) values of the bladder, rectum, sigmoid, and bowel for the plans of HR-CTV volume size of 50-60 cm3 between the 3D-printed and Rotterdam three-tube groups
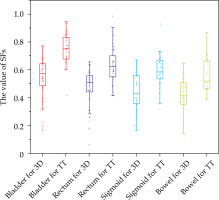
Fig. 6
Box plots of the comparison results of planning parameters homogeneity index (HI) and conformation number (CN) for the plans of HR-CTV volume size of 50-60 cm3 between the 3D-printed and Rotterdam threetube groups
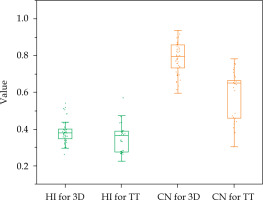
In Figure 3, for the HR-CTV category of 50-60 cm3 in the 3D-printed group, the D90 and D98 of HR-CTV were 0.35 and 0.3 Gy higher than those of the TT group (p < 0.05). In Figure 4, the average D2cc of the bladder, rectum, sigmoid, and bowel in the 3D-printed group was lower with approximately 1.3, 0.9, 0.9, and 0.8 Gy compared with those of the TT group, respectively (p < 0.05).
From the results above, when HR-CTV was < 50 cm3, the target coverage in the 3D-printed group showed obvious advantages compared with that of the TT group; although with no significant difference in the protection of OARs. However, when the volume was between 50 and 60 cm3, obvious advantages were observed both in terms of target coverage and protection of OARs. At > 60 cm3, the coverage of the target and protection of OARs in the two groups were poorer compared with those of the 50-60 cm3 category. Therefore, in comparing the results between the two groups, only the HR-CTV of 50-60 cm3 category in the 3D-printed group showed significantly better results than that of the TT group. This shows that 3D printing technique is more suitable for patients with target volume between 50 and 60 cm3.
Discussion
In our study, the dosimetry and plan parameters of patients undergoing IC/ISBT with 3D-printed interpolation technology were collected. Dosimetric differences of IC/ISBT and ICBT alone according to different target volume categories were compared. In 100 patients selected to participate in the study, the volume range of the target was large, the smallest volume was less than 20 cm3, and the largest volume was more than 100 cm3. For patients in the IC + ISBT group using 3D-printed templates, this was due to an obvious effect of tumor regression in the first two or three treatment times, since we did not change the applicator for the last treatment. For patients with TT applicators, there were patients who were unwilling to choose a relative traumatic treatment applicator, or in some patients with large volume of the target, it was relatively accepted. Therefore, we did not strictly follow the American Brachytherapy Society (ABS) guidelines [22] in the selection of applicators for patients before treatment. That explain our results to be different from those of Han et al., which showed that the target volume was greater than 30 cm3 for IC + ISBT therapy [23], while our results demonstrated that the effect was best in the 50-60 cm3 volume, which decreases beyond 60 cm3. Mohamed reported that in simple ICBT treatment, the target dose mainly comes from the uterine cavity and vaulted tube [24]. Although the dose distribution is symmetrical, it is difficult to achieve the ideal target covered while ensuring the OAR dose limit for large tumors or tumors that grow on one side, resulting in a higher rate of tumor recurrence. For our study, when the target volume was larger than 60 cm3, it resulted in all the cases above. This shows that locally advanced cervical cancer has the characteristics of large volume, eccentric growth, parametrial tissue infiltration, or metastasis, and ICBT alone cannot reach the ideal dose distribution [22]. As presented in Figure 2, the dose of IC+ISBT in the 3D-printed group was more concentrated on the prescription dose (6.5 Gy), while the dose distribution in the ICBT TT group was diffused. Meanwhile, the TT group had more abnormal discrete points (Figures 3-6).
Khanolkar et al. conducted a study on the assessment of prostate gland volume for prostate brachytherapy [25]. Their findings suggest that dedicated pre-operative volume studies are unlikely to alter management in patients with glandular volumes < 50 cm3. However, patients with cut-off score above this volume may benefit from the use of additional volumetric assessments to better characterize glandular volume and determine eligibility for brachytherapy. The current study also illustrates the correlation between brachytherapy and volume of the prostate, which is similar to our volume categories study on the correlation between brachytherapy and gynecological cervical cancer target volume.
In addition to the dosimetry comparative study between the two treatment applicators, our findings were comparable to those reported by Ren et al., who used CN and SF parameters to evaluate the plan quality of the two groups [20]. Oku et al. also used HI and CN as the evaluation quality parameters of the plans in a study on changes in the position and distance of OARs or applicators between fractions of intra-cavitary cervical cancer brachytherapy [21]. Abdalvand et al. used CN as one of the plan evaluation parameters for a model on machine learning prediction of brachytherapy for cervical cancer [26]. The unit of CN value represented the ideal situation, indicating HR-CTV completely covered by the reference dose, and that no doses larger than the reference dose were administered to normal tissues surrounding HR-CTV.
Pötter et al. reported that when performing CT scanning with an applicator, the reference of MR images was beneficial to improve the accuracy of target delineation [27]. However, the target delineation was determined in our study, but only referred to MR images and not an MR scan for target delineation with applicators in patients. Second, for the determination of target volume, we did not subtract the part containing the moulds. We found that for most of the plans, the HR-CTV did not intersect with the moulds. Therefore, for our study, the effect of the applicator on the volume of the target HR-CTV was not as great as that reported by Pötter et al.
In terms of evaluation of the total dose for the internal and external irradiation plans, we simply adopted the full parameter addition (FPA) method, which is based on the assumption that hot spots occur in the same anatomic position in each HDR fraction. We added up all the fractions of the internal and external irradiation directly, and did not, unlike Xu et al., perform the registration and superposition of internal and external irradiation [14]. Xu et al. showed that when compared with the direct addition method, OARs of the registration superposition method were lower, with no statistical difference. Similarly, Zhao et al. reported no statistical difference in the D2cc of OARs between deformed image registration and direct addition [28]. This indicates that it is reasonable to evaluate the OAR dose in our study. Harmon et al. mentioned that the expansion of the bladder has a greater impact on the dosimetry of OARs, especially for the sigmoid and bowel, in cervical cancer brachytherapy [29]. In our study, the bladder was emptied first, and then 100 ml of normal saline was injected. Therefore, our assessment of OAR dose is very accurate.
In our study, when we selected patients for 3D printing, we did not make a standard applicator virtual plan for patients, unlike Logar et al., but our findings are similar to those of Logar et al. [15]. With the use of 3D-printed individualized applicators, the target coverage was significantly improved, implying that HR-CTV D90 and D98 were significantly improved, while OARs did not exceed the dose limit. About the process of production of 3D-printed templates, our findings were different from Kut et al. [16], who combined MR and ultrasound images and considered patients anatomical information to create the templates. The 3D-printed templates, which we used to insert the needles, are only an oval mould containing one uterine cavity channel, and could only be partially individualized, compared with those by Kut et al.
In some cases, there are various practical restrictions, including poor stretchability of the vagina, highly cohesive or hard tumor tissue, too soft target, and difficulty in placing the applicator into human body, particularly in 3D printing technology. Secondly, the price is relatively expensive, and compared with the fixed applicator, it is a destructive surgical treatment, which as a process is more meticulous, cumbersome, and time-consuming, requiring a higher aseptic environment. Thirdly, some patients pain threshold is low, thus cannot withstand the pain of the procedure, and are unable to cooperate with the doctor. Under these circumstances, some patients were unsuitable for the 3D printing technique. In summary, we need to comprehensively evaluate patient suitability for the 3D printing technique, and determine what type of technology, including intra-cavity, interstitial, intra-cavity combined with interstitial, or free-hand interpolation, is the best option for the patient.
Generally, the 3D-printed template making process is relatively time-consuming, complicated, and usually takes 3 to 7 days. For patients whose tumors are progressing relatively quickly, such a long wait may not be the optimal solution. Therefore, improving the production efficiency of 3D printing phantoms will be greatly beneficial. Ricotti et al. mentioned that in the era of personalized treatment, radiation therapy may benefit from 3D printing technique [30]. Afterall, 3D printing has become an important clinical technology that can customize applicator and template designs, representing a significant progress in the implantation and delivery methods of gynecological brachytherapy treatment [31]. Desktop low-cost 3D printers are a promising solution for customized HDR brachytherapy applications [15]. In summary, for patients with locally advanced cervical cancer, image-guided IC/ISBT has advantages in both dosimetry and clinical efficacy, including reduced adverse reactions after radiotherapy, which increase both local control and survival time. With the development of imaging technology, application of 3D printing technology and improvement of applicators, the rapid development of IC/ISBT will need further evaluation [30-33].
Conclusions
In the IC + ISBT group compared with the ICBT TT treatment group, the target coverage, OARs protection, and planning parameters based on 3D-printed template showed more advantages, especially for those plans with target volume between 50 and 60 cm3 in locally advanced cervical cancer patients. This implies that for patients with target volume ranging from 50 to 60 cm3, it is more suitable to use IC + ISBT 3D-printed applicator. We hope that our study can provide a clinical reference for HDR brachytherapy in locally advanced cervical cancer.