Purpose
Nasopharyngeal carcinoma (NPC) is the most common cancer originating in nasopharynx. It is vastly more common in certain regions of East Asia and Africa than somewhere else, with viral, dietary, and genetic factors implicated in its causation [1,2,3,4,5]. Radiotherapy is the primary and radical therapy for non-metastatic NPC [2,3,4,5,6,7,8,9,10,11,12]. However, 15-48% of NPC patients still suffer local failure (persistence and recurrence) after radical radiotherapy. Although re-irradiation is an established form of salvage treatment, with local control rates of 22-80% and further 5-year survival rates of 14-82%, high-dose re-irradiation provided by conventional external beam radiotherapy (EBRT) may assume higher risk of treatment toxicity [13]. For residual tumor with deep invasion, the results of salvage treatment for these NPC patients remain unsatisfactory [14,15,16,17,18]. Therapeutic approach should identify a treatment that gives the greatest control rates and the best quality of life after therapy. However, potential side effects of treatments must be considered along with potential benefits. Brachytherapy may play a meaningful role in the treatment of residual NPC because it provides advantages over EBRT – the tumor can be treated with very high dosage of localized radiation, while reducing the probability of unnecessary damage to surrounding healthy tissues [12,15,16,19]. In this paper, we propose a technique using fibre-optic endoscope-guided three-dimensional high-dose-rate interstitial brachytherapy (3D HDR ISBT) boost to treat deep-seated residual lesion of NPC after conventional radical EBRT.
Case description
In January 2016, a 52-year-old female sought medical attention at our institution after being diagnosed with hearing loss involving left ear. After routine diagnostic examination, she had an abnormal magnetic resonance imaging (MRI) scan revealing an area of distortion of approximately 3.9 × 2.2 × 2.6 cm3 in size, which invaded the left postero-lateral nasopharynx and filled the pharyngeal recess (Figure 1). The nasal endoscopy-guided biopsy of nasopharyngeal mass demonstrated low-differentiated squamous cell carcinoma (SCC). She was diagnosed with SCC of left nasopharynx (T2N0M0; II stage according to the 7th edition of the American Joint Committee on Cancer, AJCC), and agreed for treatment consisted of radical radiotherapy and concurrent chemotherapy.
Treatment modalities
The patient received EBRT using intensity-modulated radiation therapy (IMRT) at the beginning of treatment. The primary tumor received a total dose of 69.96 Gy in 33 fractions (2.12 Gy/fraction/day). High-risk clinical target volume that was defined as primary tumor plus a 10 mm margin to encompass high-risk sites of microscopic extension, and the whole nasopharynx received an accumulated radiation dose of 59.40 Gy in 33 fractions (1.80 Gy/fraction/day). The total dose to clinically uninvolved areas of the neck was 50.40 Gy in 28 fractions (1.80 Gy/fraction/day), including supraclavicular fossae (Figure 2). Chemotherapy was given with intravenous cisplatin 100 mg/m2 every 3 weeks for 3 cycles, concurrently to EBRT. After chemoradiotherapy, the tumor size decreased to 3.3 × 1.8 × 2.2 cm3 according to the MRI examination, which was performed immediately after the treatment. The doses received by organs at risk (OARs, including spinal cord, brain stem, optic nerve, optic chiasm, temporal lobe, temporomandibular joint, pituitary, nasal septum, and soft palate) approached the maximum tolerated dose of normal tissues. Three months after the completion of initial chemoradiotherapy, the MRI scan showed an irregular mass (persistent disease with same size as 3 months before) near to left nasopharyngeal roof and posterior nasal cavity (Figure 3A). A diagnostic biopsy remained positive and revealed a locoregional residual nasopharyngeal SCC. Despite recommendation for subsequent external beam boost or nasopharyngectomy integrated into salvage treatment plan, the patient refused external beam re-irradiation or surgery because of the possibility of severe treatment toxicity related to high cumulative radiation dose with EBRT and high incidence of complication by radical surgery. The patient accepted brachytherapy as salvage treatment.
Fig. 3
A) Locoregional residual tumor 3 months after completion of EBRT. B) Two needle applicators inserted to the target under fibre-optic endoscope guidance. C) CT images of applicator spatial location after implantation
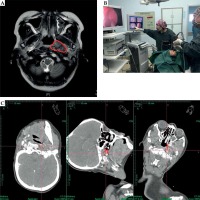
Before processing, an informed consent was obtained from the patient, and all procedures were performed with standard institutional approval. The applicators with titanium interstitial needles (Nucletron, an Elekta company, Elekta AB, Stockholm, Sweden) were used, and the insertion and treatment planning were performed before each treatment session. Fibre-optic endoscope-guided 3D HDR ISBT boost, with a total dose of 14 Gy in 2 fractions (7 Gy/fraction) and interval between sessions of 7 days (once per week) was delivered; deep-seated residual NPC was located 3.3 cm below nasopharyngeal epithelium. The insertion procedure was performed under general anesthesia, with electrocardiogram, arterial oxygen pressure, respiration, and blood pressure monitoring. Under the guidance of fibre-optic endoscope, two needle applicators (1.9 mm in external diameter and 240 mm in length) were angle-bended (less than 30 degrees to avoid obstruction of radiation source), and inserted through the nose into the residual tumor of left nasopharynx in a way to facilitate irradiation of the whole tumor area and simultaneously control the position of needles against adjacent structures using image guidance system (Medtronic Navigation Inc., Louisville, USA) (Figure 3B). The needles with an appropriate implantation pathway and insertion depth for interstitial treatment were placed into the treatment volume, with referencing to MRI images before ISBT (Figure 3C). The needle applicators were positioned in such a way that their layout in the lesion was possibly most parallel, and their distance from one another was 1 cm to ensure adequate dose distribution and target volume coverage. At the end of the procedure, biodegradable synthetic polyurethane foams packing NasoPore (Polyganics BV, Groningen, The Netherlands) was applied to nasopharyngeal cavity, followed by immobilization of the needle applicators using a button sewed into the wings of the nose. Once the needle applicators were in place, they remained in the lesion throughout the treatment process and were removed after each treatment session. After implantation, fine-pitch (2 mm) X-ray computed tomography (CT) images were acquired and transferred to treatment planning computer. CT imaging data was fused with previously performed MRI examination and used to contour target volume for 3D HDR ISBT. GTVISBT was defined as residual malignancy. As a dose evaluation reference, CTVISBT was defined as GTVISBT plus a 10 mm margin in lateral directions and restricted by the volume of OARs to encompass high-risk sites of microscopic extension, according to the tumor range before EBRT. A CT-based treatment plan was created using a graphic optimization tool (Oncentra v. 4.3; Nucletron, an Elekta company, Elekta AB, Stockholm, Sweden) (Figure 4). Normalization and optimization of the target volume was performed, and time and location of ionizing radiation source stopping points were planned.
Fig. 4
Dose distribution of horizontal, sagittal, and coronal positions in the first 3D HDR ISBT irradiation session
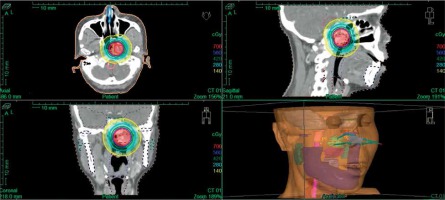
Dosimetry parameters were reported according to guidelines. The dose volume histogram (DVH) parameters were recommended for the evaluation of target volume and OARs (Figure 5). In order to compare EBRT and ISBT with conventional fractioning of 2 Gy, the doses were converted to dose equivalent in 2 Gy fractions (EQD2 model), with α/β = 3 (GyEQD2, α/β = 3) for OARs and α/β = 10 (GyEQD2, α/β = 10) for target. DVH parameters were analyzed taking into account the target volume receiving 90%, 100%, 150%, or 200% of PD (V90%, V100%, V150%, and V200%, respectively) and the equivalent dose delivered to 100%, 98%, 90%, and 50% of the target volume (D100%, D98%, D90%, and D50%, respectively). These doses were converted according to linear quadratic model of biologic effective dose (BED). BED and EQD2 were calculated for each fraction and total fractions. Due to various locations, the maximum doses received by OARs were reported. The doses were converted to BED and to EQD2 according to the linear quadratic model.
Fig. 5
DVH parameters in the first 3D HDR ISBT irradiation session. High-dose distribution for target volume and low-dose distribution for OARs are shown
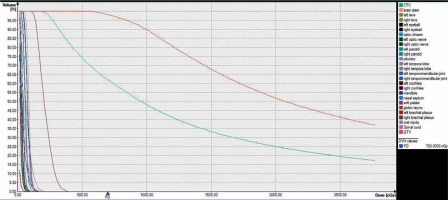
The treatment was conducted using 18-channel iridium-192 (192Ir) remote afterloader system (Microselectron HDR 192Ir; Nucletron, an Elekta company, Elekta AB, Stockholm, Sweden). After the completion of ISBT, the applicators were removed and nasopharyngeal cavity was packed with absorbable gelfoam in order to prevent post-operative bleeding. CT scan with contrast was performed, and after 2 hours of observation to rule out potential complications, the patient was discharged. The patient was required to regularly attend follow-up visits at our affiliated clinics.
Results
In spite of slight respiratory movement of anatomic structures in head and neck region, image-guided insertion of the needle applicators was precise and relatively safe, even with close vicinity of many OARs. According to DVH parameters, the actual doses achieved in 3D HDR ISBT plan are characterized in Table 1. Depending on the location of irradiated area, the total doses received by OARs were as follows: Dmax-spinal cord was 2.79 GyEQD2, Dmax-brain stem was 5.38 GyEQD2, Dmax-left optic nerve was 1.10 GyEQD2, Dmax-right optic nerve was 0.75 GyEQD2, Dmax-optic chiasm was 1.83 GyEQD2, Dmax-left temporal lobe was 5.11 GyEQD2, Dmax-right temporal lobe was 1.84 GyEQD2, Dmax-left temporomandibular joint was 2.28 GyEQD2, Dmax-right temporomandibular joint was 0.64 GyEQD2,Dmax-pituitary was 2.14 GyEQD2, Dmax-nasal septum was 0.48 GyEQD2, and Dmax-soft palate was 7.64 GyEQD2.
Table 1
Doses (Gy) of D100%/98%/90%/50%, BED/EQD2-D100%/98%/90%/50%, and value of V90%/100%/150%/200%
[i] D100%/98%/90%/50% – dose covering 100%, 98%, 90%, and 50% of target volume, V90%/100%/150%/200% – target volume (percentage and cm3) receiving 90%, 100%, 150%, and 200% of prescription dose, BED-D100%/98%/90%/50% – biologic effective dose in D100%, D98%, D90%, and D50%, EQD2-D100%/98%/90%/50% – dose equivalent 2 Gy in D100%, D98%, D90%, and D50%, Fr – fractions
No complications were reported during the treatment and 3D HDR ISBT was well tolerated by the patient. The radiotherapy-related toxicity was measured using the Radiation Therapy Oncology Group (RTOG) scale. Before 3D HDR ISBT, radiation-induced skin reaction (RTOG scale grade 1) was be observed with redness and pigmentation, and salivary glands reaction (RTOG scale grade 2) with moderate xerostomia. The target volume at the time of the last treatment session of 3D HDR ISBT reduced from 3.3 × 1.8 × 2.2 cm3 (before ISBT) to 3.0 × 1.6 × 1.9 cm3 without additional adverse reactions. Nearly 3 months after completion of 3D HDR ISBT, residual NPC shrank in size to 2.9 × 1.5 × 1.6 cm3. During follow-up examination in the 6th month after 3D HDR ISBT, the size of refractory residual tumor in left nasopharynx reduced to 2.6 × 1.3 × 1.5 cm3, according to the MRI examination. Within 12 months after 3D HDR ISBT boost, the residual tumor has completely regressed. The treatment-related skin side effects have completely healed, and xerostomia disappeared. At the present 26 months after salvage treatment, there are no signs of complications, nor evidence of recurrence at the site of 3D HDR ISBT.
Discussion
Locally persistent NPC remains a challenge because is associated with risk of local recurrence, if satisfactory additional treatment is not applied. Kwong et al. [8] assessed 803 patients with non-metastatic NPC, who completed a full course of radical EBRT, and 55 (6.9%) patients with persistent positive biopsies beyond 12 weeks post-radiotherapy had a 40% local control at 5 years. Authors concluded that the failure of treatment was related to the residual lesion. Some studies determined that maximizing local control was crucial for the survival of patients with locally persistent NPC [15]. It is a remarkable fact that early detection of local failure and adequate total dose by re-irradiation were vital for maximizing the chance for local salvage [20,21,22,23]. In a study investigating a cut-off after radiotherapy to define persistent disease, 12 weeks of continuous positive histology after EBRT was considered as persistent disease and additional salvage treatment was initiated [8]. Also, it has been emphasized that radiation boost to residual tumor sites should be withheld unless the biopsy remained positive at 10 or more weeks after EBRT [12]. A retrospective analysis by Lee et al. [9] determined that high-dose re-irradiation with EBRT could achieve successful local salvage in substantial number of local failure NPC patients, but the incidence of severe side effects and late complications might increase significantly, and influence the course of treatment and quality of life. Chua et al. [23] compared treatment outcomes in patients with local failure NPC receiving re-irradiation by EBRT or brachytherapy. In patients with EBRT, the median 3-year and 5-year performance-adjusted survival (PAS) rates were considerably worse than in patients receiving brachytherapy as salvage treatment. In addition, all major complications were more common in patients re-treated with EBRT than those re-treated with brachytherapy.
Some exploratory studies have focused on application of brachytherapy in the treatment of head and neck cancers, and observational results confirmed that combination of EBRT and brachytherapy should be considered whenever feasible and large fractional dose avoided to minimize late complications [10,17]. Leung et al. [16] investigated salvaged HDR intracavitary brachytherapy (ICBT) boost in patients with locally persistent NPC. They found that adding HDR ICBT radiation boost to EBRT was effective in treatment of persistent NPC, as shown by a statistically significant improvement in local failure-free survival, disease specific survival, and overall survival rates at 5 years with acceptable toxicity. However, it was unknown whether the residual tumor volume extended beyond the prescribed depth of ICBT. Wan et al. [12] evaluated treatment outcomes with implementation of brachytherapy radiation boost for residual NPC. The dose and survival advantages suggested that the ISBT boost might be a promising selection for a deep-seated NPC residual lesion (especially for more than 1 cm below the nasopharyngeal epithelium). In addition, rapid dose fall-off beyond the target volume with the use of brachytherapy minimizes radiation to surrounding OARs, and patients receiving such treatment developed fewer complications and had better performance, as compared with EBRT [23].
In the present case, the fibre-optic endoscope-guided 3D HDR ISBT boost was used in our patient to control deep-seated residual NPC after radical EBRT. DVH with satisfactory dosage distribution showed that the contours of target volume were covered with high-dose irradiation completely and doses to OARs were relatively low. Importantly, the fibre-optic endoscope-guided 3D HDR ISBT had a limited dosage in paranasopharyngeal vital organs, and evidently reduced the risk of radiation-induced tissue necrosis and dysfunction. Furthermore, when combined with EBRT, the total dosage was easily elevated to more than 70 Gy for the tumor. The NPC patient with residual lesion obtained good loco-regional control by 3D HDR ISBT with fibre-optic endoscope guidance. The tumor shrank visibly within 12 months, and no serious complications were found in follow-up over 2 years. Positive effects were achieved in case of both treatment efficiency and quality of life.
Conclusions
Despite an ongoing discussion regarding the best management and/or optimal treatment procedure for locally residual NPC (especially with deep-seated lesion), the fibre-optic endoscope-guided 3D HDR ISBT boost may be a valuable salvage approach with tolerable toxicity in selected patients. In addition, whenever possible, this treatment should be integrated with EBRT, depending on the clinical response after high-dose EBRT and availability of expertise.