Purpose
Esophageal cancer is one of the fastest growing and deadliest cancers in the world. During the past three decades, cancers originating in the lower esophagus have increased by 600% [1]. In Western countries, it is this adenocarcinoma form that develops in the distal esophagus that primarily accounts for the increase [2]. In 2018, the estimated incidence of esophageal cancer in the United States was 17,290 cases, and the estimated number of deaths was 15,850 [3]. Worldwide, esophageal cancer is the 8th most common cancer, with an estimated 572,034 new cases and 508,585 deaths predicted in 2018, making it the world’s 6th deadliest cancer [4]. Earlier diagnosis and innovative therapies for esophageal cancer treatment are dearly needed.
For esophageal cancer patients treated with a non-operative approach, phase III trials using concurrent chemoradiation therapy with external beam radiation doses of 50-64 Gy have revealed suboptimal tumor control with high, crude local failure rates of 45-55% [5, 6]. Further dose escalation with external beam radiation therapy to improve these failure rates seems improbable since the nearby, radiosensitive organs at risk, such as the lungs, heart, trachea, major bronchi, and spinal cord, limit the amount of external radiation that can be delivered safely.
Intraluminal high-dose-rate (HDR) brachytherapy is an efficient method of delivering high doses to esophageal tumor with adequate spatial precision as a boost after external beam, or as a primary therapy for early-stage, localized esophageal cancer [7, 8]. According to the American Brachytherapy Society (ABS) consensus guidelines for brachytherapy of esophageal cancer, relative to external beam radiation therapy (EBRT), brachytherapy offers the potential advantage of increasing the dose to esophageal tumor while delivering a relatively low-dose to the surrounding normal tissues, particularly the lung, spinal cord, and adjacent normal esophageal mucosa [9]. However, concerns have been raised that the rapid dose fall-off seen in a standard single-channel intraluminal technique has disadvantages, as the dose delivered to the mucosal surface is much higher than that delivered to the prescription depth [10] as well as that brachytherapy may lead to an unacceptable incidence of acute and chronic esophageal ulcers, fistulas, or stricture formation, particularly when combined with concurrent chemotherapy and EBRT [11].
Institutions have investigated HDR brachytherapy in early-stage esophageal cancer patients as a boost after external beam radiation or as a primary therapy. Tamaki and colleagues demonstrated a local control rate of 79% at 5 years for 54 patients undergoing external beam radiation therapy, followed by 2 or 3 fractions of brachytherapy [12]. A prospective, phase I/II Radiation Therapy Oncology Group (RTOG) trial was completed using five weeks of external beam radiation with chemotherapy, followed by three fractions of HDR brachytherapy [11]. Unfortunately, a high-rate of treatment toxicity, including a 1-year actuarial fistula rate of 18%, was reported using a standard, single brachytherapy catheter technique. Two recent reviews have examined the clinical literature on the role of brachytherapy in early-stage esophageal cancer [13] and in palliative treatment for esophageal cancer [14]. In the first review, twelve studies involving 514 patients with stage 1 cancer were analyzed for local control, disease-free survival, and overall survival, following brachytherapy (low-dose-rate [LDR] and HDR) with or without external beam therapy. They concluded that the combination therapy was effective enough to be considered an alternative to surgery. In the other review, seven randomized studies with 905 patients were included. The authors concluded that brachytherapy improved both dysphagia-free survival and quality of life compared to other treatments (external beam radiation, photodynamic therapy, stenting, laser, and argon plasma coagulation). The most recent data in these reviews showed quite variable toxicity, ranging up to 23%.
Esophageal HDR treatments have typically been delivered using a single-catheter with the dose prescribed to a depth of 5-10 mm. Because of the uncertainty of the position of a single brachytherapy catheter relative to the esophageal tumor target volume, we had previously developed a novel three-catheter technique that improved the dose homogeneity and normal tissue maximum doses [15]. We have now improved our esophageal brachytherapy applicator design by inventing a balloon repositioning, multichannel applicator using 5 or 6 peripheral channels that extend along the length of the applicator over a therapeutic balloon, and terminate at the midpoint of an anchor balloon. Our goal is to optimize dose coverage for a three-dimensional tumor target by modulating the brachytherapy dose within these 5 or 6 channels. The therapeutic and distal anchor balloons of this applicator may be filled with water or air to displace the channels up against the esophageal wall/tumor.
In this study, we evaluated the three-dimensional radiation dose distribution of this new multi-channel esophageal applicator prototype with either air or water filled therapeutic balloon. Additionally, we investigated improvements in three-dimensional dose mapping by increasing the number of channels available for three-dimensional planning. We theorize that this new applicator will allow better conformality of dose to the target, and lower doses within the healthy, normal tissues, potentially allowing for safe brachytherapy dose escalation. The studies presented here were done on a prototype, which we envision will lead to clinical use and possibly, a multi-center trial.
Dose calculations for 192Ir HDR brachytherapy typically assume a water equivalent medium. However, it has been shown that for HDR treatments of the esophagus, ignoring the effects of nearby bony structures and air in the trachea, can lead to a 15% error in dose estimation in various organs at risk [16]. Since, the applicator described in this work will most likely be inflated with air and not water due to weight considerations, we have used a model-based computations available in Oncentra TPS to evaluate the effect of that heterogeneity.
Material and methods
A new inflatable, repositionable, multi-channel applicator for esophageal HDR brachytherapy has been developed in the polymer core laboratory in collaboration with the Cleveland Clinic Medical Device Solutions Team. The applicator has two inflatable balloons: a spherical, anchor balloon located just proximal to a semi-hard distal tip, and a cylindrical, therapeutic balloon located proximal to the anchor balloon. The balloons are made of silicone and nylon. On the periphery of the therapeutic balloon are six LumenCare® applicators (Elekta AB, Stockholm, Sweden). The applicator is both CT and MRI compatible. Both the anchor and therapeutic balloons can be inflated with water or air. For the film dosimetry measurements, 80 ml of air or 50 ml of water were introduced into the therapeutic balloon, respectively, using a standard syringe. A simple water phantom was constructed using a rectangular plastic bin filled with 45 liters of distilled water, and containing several slabs of solid water, onto which film and the applicator could be taped. Figure 1A is a photo of the water phantom with the multi-channel applicator taped to a slab of solid. Figure 1B shows two computed tomography (CT) cross-sections of the applicator, one water-filled and the other air-filled. Channels were loaded with guide wires constructed with a train of dummy seeds in order to be identified in CT images. Various channel loadings were used to demonstrate lateral directionality and dose modulation possibilities of this applicator. CT image sets were obtained with our clinical scanner (Phillips Big Bore®, Philips North America Corporation, Andover, MA, USA). CT-based planning was performed with the Oncentra (Elekta) treatment planning system (TPS) v. 4.5, based on TG43 formalism. For TG-186 calculations [17], air in the therapeutic balloon was manually contoured and set to its default density.
Fig. 1
A) Photograph from above the water phantom with the applicator and film in place; B) CT images of the applicator with a water-inflated (left) and an air-inflated (right) treatment balloon
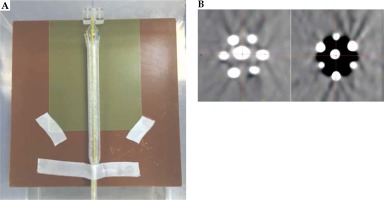
For the film data reported here, 400 cGy was prescribed to dose points, 1 cm away from the applicator surface. Dose was delivered from all 6 channels using MicroSelectron v. 3 (Elekta AB, Stockholm, Sweden) afterloader. A piece of Gafchromic EBT3 film (Ashland Advanced Materials, Bridgewater, NJ, USA) was placed at the prescribed plane as well as directly next to the applicator, respectively, in order to measure planar dose distributions. Films were scanned at least 24 hours later on an Epson Perfection 4990 flatbed scanner (Epson America, Inc., Long Beach, CA, USA); a film calibration curve was established by exposing film to 15 different doses in the range of 20 cGy to 1000 cGy from a TG-51 calibrated 6 MV linac. Film and TPS generated dose distributions were analyzed using IBA’s OmniPro-I’mRT software (IBA USA, Reston, VA, USA). Scanned transverse dose profiles were compared (measured vs. Oncentra calculated), and gamma analysis was performed with a 3%/3 mm criterion.
Dose modulation was examined using several simulated treatment cases designed to include a range of what might be found in clinical practice: 5 mm thick ring target around the applicator, a 5 mm thick target consisting of approximately 25% of the ring circumference, and a ring target of two thicknesses (5 and 2 mm) of approximately 50% of the ring circumference. Oncentra image registration tools were used to construct the targets. For all three examples, treatment plans were done to compare dose distributions between utilizing 6 or 3 peripheral channels and a simulated single central channel. Graphical optimization was used to shape the dose distribution for multi-catheter plans. Note that this device does not currently have a single central channel; therefore, the central rod was digitized as if it were a usable source channel. Dose distributions shown in Figures 2A and 3A, B were calculated using the TG-43 formalism. Dose volume histograms were obtained using default settings in the treatment planning system.
Results
TG-43 and TG-186 dose distribution
For commissioning of the TG-186 algorithm (ACE), three test cases from the Brachytherapy Source Registry (IROC, Houston, TX, USA) containing air and water were downloaded and the dose points calculated to be within an average of 1.8% of expected values [18].
A dose distribution comparison between the TG-43 Oncentra-generated and the film-measured distribution for a coronal plane at the applicator surface is shown in Figure 4. Results for a water-inflated applicator are presented in Figure 4A, using a dose grid of 1 mm. A dose profile comparison in X direction and a gamma plot for the data were included. There was a small difference at the peak value; gamma analysis resulted in 99.84% of the pixels passing the 3%/3 mm criterion. When air was used for inflation in place of water, the TG-43 calculated and measured dose distributions did not agree as well (Figure 4B). Gamma analysis had only 80.8% of pixels passing the same criterion as above. The dose difference at the peak value was also increased.
Fig. 4
2D dose profiles comparison showing the calculated dose (green) and the measured (red) dose and 2-d planar dose differences for A) water-filled balloon, TG-43 calculation; B) air-filled balloon, TG-43 calculation; C) air-filled balloon, TG-186 calculation
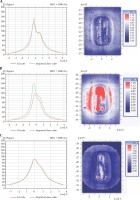
When TG-186 calculations were used, the Oncentra and film results were much more similar for the air-filled therapeutic balloon (Figure 4C). The gamma analysis had 99.53% of pixels meeting the 3%/3 mm criterion. The peak dose values were very similar.
Dose comparisons were also made between TG-43 and TG-186 for a non-circumferential target (25%) described above. The axial distribution in a plane approximately halfway down the target using TG-43 is shown in Figure 2A; for TG-186, it is in Figure 2B. They appear to be very similar. Dose volume analysis confirmed the similarity. Implant volumes in the range from V50 to V200 were within 2% of each other in TG-43 vs. TG-186 comparisons. TG-43 values were consistently slightly higher. The contralateral surface had a dose difference of about 3% (60% vs. 62% of prescription). Dose distributions in the next section are for TG-43-based calculations.
Single-centered catheter versus multiple peripheral catheters
Figure 3 shows the cross-sectional dose distributions for a target ring of 5 mm thickness, using one central channel (A) or 6 peripheral channels (B). The dose was normalized to 5 mm depth from the applicator surface; source dwell points were separated by 5 mm in each channel, using optimization on dose points. Figure 5 is an example of using all 6 channels for a target having 2 thicknesses. Graphical optimization was applied to conform the 100% isodose line to the target depths. If a single central catheter was used, the dose distribution was as shown in Figure 3A for any of the cases discussed. The volume data provided in Table 1 were taken from dose-volume histogram (DVH) analysis from Oncentra software. The non-target V100 was the volume of 5 mm ring enclosed by the 100% isodose that was not a part of the target. It was obtained by subtraction of V100 values from the ring volume (16.6 cc). These data were used to calculate a conformity index (CI = TV/PTV; ICRU 62), for which the treatment volume (TV) was the volume of tissue re-ceiving 100% dose (it obviously excludes the applicator) and the contoured target was taken as the planning target volume (PTV).
Fig. 5
Central cross-sectional planar dose distribution showing 2 targets treated with 6 catheters. Targets are yellow and brown. Isolines shown are as in Figure 2 (no 60%)
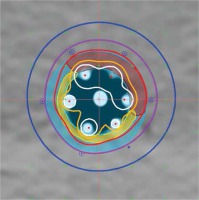
Table 1
Dose-volume comparison between the multi-channel and single channel applicators for 3 cases
For the single central catheter, the dose at the applicator surface was approximately 170%, at 5 mm, 100%, and at 10 mm from the surface, it was 71%. For the 3-catheter example, these values were 200%, 100%, and 33%, and the contralateral surface was 60%. For the 6-catheter case, the corresponding doses were 200%, 100%, and 50%.
Discussion
We investigated the effect of the presence of air within the therapeutic balloon compared to water on the dose distribution. This has been discussed with recommendations published [17] in regard to brachytherapy. Our data support these recommendations in that measured dose distributions agree very well with model-based dose calculations. The effect of going from TG-43 to TG-186 was modest. Implant V100 and V150 values changed about 2% in the case of using all 6 channels. In the 3-channel case, the changes were slightly larger (3% to 5%). This is likely due to the fact that full scatter was provided on the tissue side of the applicator.
Similar results have been reported by others [18]. In a retrospective dosimetric comparison for 38 accelerated partial breast irradiation patients [19], small but significant changes in clinically relevant DVH parameters were seen when TG-186 calculations were used in place of TG-43 on Oncentra planning platform. In another recent study [20], it was suggested that ACE may underestimate dose to bone by as much as 10%, and that TG-43 might be better for dose calculations in scalp brachytherapy. It appears that the clinical usefulness and significance of TG-186 calculation should be evaluated for specific sites and applicators.
The purpose for the design of this applicator was to provide asymmetry in the dose distribution, so as to be able to conform dose coverage to targets that vary in size and position. Clearly, a single-catheter only provides a symmetric distribution in the radial direction, therefore, may overdose some parts of the esophagus in order to cover the greatest extent of the disease. Single-catheter applicators, unless equipped with centering devices, may change position radially resulting in overdosing and underdosing different regions. Further, even with a centering device, a single central catheter cannot modulate dose to a tumor radially, which may have different thicknesses at different locations around the diameter and along the length of the esophagus. The anchor and therapeutic balloons along with 6 peripheral channels were designed to minimize such positional effects while offering the possibility of improving dose conformity to the target. Data presented in Table 1 indicate that for partial ring targets, this applicator has superior sparing of non-target tissue. Thus, for the 1 vs. 3 catheter case, the sparing was 10-3.4 – 6.6 cc (66%), and for the 1 vs. 6 case, it was 6.6-1.7 – 4.9 cc (75%). Target coverage was maintained in all cases (99%). The conformity index also reflected better conformity to the target improvement, 2.5 vs. 1.5 (40%) and 1.7 vs. 1.2 (30%).
The dose estimates for the simulated single-catheter case are in agreement with published data for a commercial applicator (no longer available it seems) that provides centering balloons [21]. A multi-channel applicator for esophageal treatments has also been described [22]. It is non-inflatable, has 10 peripheral channels, and allows insertion of endoscope in central channel. The main advantage of the one described here is its inflatability to different diameters. The discussed dosimetric examples were for a 40 ml air inflation yielding a 1 cm applicator radius. Greater or lesser inflations are possible. Because of the geometry of our applicator and the proximity of source dwell positions to the applicator surface, the maximum doses to surrounding tissue may be considerably higher than for a single-centered catheter applicator; although, that may be less for a catheter without a centering device. Prescription doses would need to be reconsidered in that light. However, it should be noted that repositioning the device from one fraction to the next and the effects of breathing motion would tend to distribute hot spots, thereby, lessening the potential damage.
The concept of inducing dose asymmetry by using several peripheral channels has been applied to commercial applicators intended for other sites. For example, a multi-channel vaginal cylinder (Elekta) and an inflatable multi-channel balloon for partial breast radiotherapy (SAVI, Cianna Medical Inc., Aliso Viejo, USA) have been available for some years. An inflatable applicator for treating rectal lesions has been described recently [23]. The concept is unusual in that the catheters all seem to be placed on one side of the inflating rectal balloon and may limit the circumferential size of the intended target.
All of the above applicators offer the possibility of reducing dose to and thus, sparing the contralateral side of the treatment site. Attention to increased surface dose at the ipsilateral side is warranted.
Conclusions
A balloon inflatable, multi-channel applicator for endoluminal HDR treatment of esophageal cancer has been developed and tested in vitro. It permits several degrees of freedom in conforming the dose distribution to non-uniform, asymmetric targets.
The use of model-based dose calculations produced only a small change in the dose distribution due to the presence of a significant air volume.