Purpose
Head and neck cancer is the sixth most common cancer worldwide [1,2]. Over 800,000 new head and neck cancer cases are diagnosed and nearly 450,000 head and neck cancer-related deaths occur annually worldwide, as of 2018 [1,2]. About two thirds of patients with head and neck carcinoma (HNC) are diagnosed at an advanced stage of the disease [3]. Surgical resection followed by radiotherapy or post-operative chemoradiation/definitive chemoradiation are employed as treatment modalities, with curative intent for patients with locally advanced disease. Unfortunately, the likelihood of treatment failure for patients with locally advanced HNC remains high, reaching 50%, with locoregional failure and distant metastases occurring in 20-30% of patients [3,4,5,6,7,8]. Locoregional failure has remained the predominant pattern of failure and it is the most common cause of death in HNC patients [9,10]. Patients with recurrent or metastatic HNC have a poor prognosis, with median overall survival of less than one year [8,11,12], necessitating a pressing need to improve therapy for patients with recurrent HNC.
Prognosis is particularly poor when the recurrence develops in an area previously treated with radiation [11,12]. Salvage surgery in previously irradiated cases can provide durable disease control in 15% of patients with locoregional recurrence in the absence of disease at distant sites [3]. Nevertheless, the pathological findings following salvage surgery frequently indicate that the patient requires a second course of post-operative radiation due to the unclear or positive surgical margins or other adverse pathologic features [3,13].
Due to technological advances in radiation oncology in the recent years, re-irradiation using external beam radiation therapy (EBRT) has become an option. Unfortunately, most studies involving EBRT for re-irradiation are retrospective and report on a small patient cohort and a single institution experience; the treatment regimens and patient characteristics vary considerably between studies. Moreover, the treatment-related toxicity may be very significant and may include surgical wound healing complications, fistula formation, osteoradionecrosis, vascular events, transverse myelitis, brainstem and cranial nerve injury, etc. [3,12,14].
Brachytherapy has significant advantages over external beam radiation therapy, thus should be considered when a second course of radiation is indicated [15]. Compared to EBRT, brachytherapy facilitates the delivery of a high and localized radiation dose to the target volume. The dose to the surroundings tissues is significantly reduced due to the characteristic rapid dose falloff [13]. The reduced radiation delivery time of brachytherapy relative to conventionally fractionated EBRT may increase the effectiveness of radiotherapy; in particular head and neck cancer is known to have relatively short radiobiologically estimated repair half-life. Furthermore, given that the hypoxic mass of the tumor is removed during salvage surgical resection, hypothetically, only well-oxygenated microscopic disease remains around the surgical margins. Therefore, a lower radiation dose could be sufficient with the use of brachytherapy [16].
The majority of the published brachytherapy results were obtained with low-dose-rate, high-dose-rate, or pulsed-dose-rate techniques and manual afterloading [17,18,19,20,21,22]. Compared to other isotopes, Cesium-131 (131Cs) is a relatively new permanent seed implant brachytherapy isotope, with an average photon energy of 30 keV and short half-life of 9.7 days [23]. Compared to external beam radiation or high-dose-rate brachytherapy using Iridium-192, the lower energy photons from 131Cs decay allow for more localized deposition of dose, decreasing the dose delivered to nearby tissues, and potentially reducing treatment complications. The feasibility of 131Cs implants for recurrent HNC has been demonstrated with acceptably low levels of exposure to staff during the procedure [24]. In a small series, re-irradiation using permanent 131Cs has been shown to provide similar rates of survival with decreased toxicity, compared to prior literature [25].
The purpose of this report is to describe a single institution’s implementation process of utilizing intraoperative, permanent 131Cs seed implants for patients with completely resected recurrent HNC. The process of pre-planning, pre-implant seed ordering, intraoperative procedures, post-implant planning, and radiation safety protocol are described.
Material and methods
Patients were treated per an institutional protocol for recurrent resectable HNC. Fifteen patients receiving 131Cs permanent seed brachytherapy were included in this analysis.
Description of the experimental product
IsoRay™ (Richland, WA) provides the sterilized Proxcelan™ 131Cs [23] seeds used in all implants in either strands or a mesh. Seeds contain an inorganic substrate adsorbed with the radioactive 131Cs isotope and a 4 mm radio-opaque gold marker encased in a 0.05 mm titanium capsule, as seen in Figure 1. The outer diameter and length of the capsule are 0.82 mm and 4.5 mm, respectively. The vendor manufactures the seeds and places them into 3-0 vicryl strands at 1 cm intervals or in a mesh with user-defined spacing and positioning. The strands or mesh can then be sutured directly into the resection bed.
Implant pre-planning and dose prescription
Tumor volumes were identified on a preoperative positron emission tomography/computed tomography (PET/CT) scan. The estimated size and location of the resection cavity were estimated by the radiation oncologist and otorhinolaryngology surgeon, and the optimal plane to suture stranded seeds or a mesh was identified. A surgical mesh is available for purchase with customizable seed and strand spacing. The constructed mesh is then provided to the institution sterilized. The decision to utilize stranded seeds or a predesigned mesh depends on surgeon preference as well as implant location. An implant that spans across the carotid artery is an example of one location that may benefit from strands of seeds instead of a mesh, allowing for strands to be individually sutured on either side of the critical structure with adequate spacing.
Using MIM Symphony LDR™ treatment planning software, version 6.5 (Cleveland, OH, USA) [26,27], the seeds were placed in a single, optimal plane with 1 cm seed-to-seed spacing to cover the estimated resection cavity. The seed air kerma strength was iteratively adjusted in the planning software, such that a dose of 60 Gy was delivered to a prescription located 5 mm perpendicular to the center of the implant plane. Planning details have previously been reported [28]. The treatment plan was reviewed by the medical physicist, radiation oncologist, and otorhinolaryngology surgeon. The prescription dose was decreased in cases where there was a concern for normal tissue toxicity by the radiation oncologist. In most cases, especially those with larger uncertainty in the size or geometry of the estimated resection cavity, an extra strand of seeds was ordered.
Seed ordering and receipt
Sources were ordered five to seven business days prior to implant date from IsoRay™ for each patient. Radioactive materials were delivered to the radiation safety office per institutional protocols, including wipe tests and source inventory recording. Sources were then transferred to the department of radiation oncology, accepted by a medical physicist, logged in the source inventory, and stored in a secure location designated for radioactive material. Non-sterile, loose seeds from the same lot as the implant seeds were ordered and assayed for verification of vendor-stated activity. The number of assayed seeds was either 10% of the number of seeds in the implant or five seeds, whichever was larger [29]. Assays were performed using an accredited dosimetry calibration laboratory-calibrated HDR 1000 Plus well-type ionization chamber and Max-4000 electrometer for each patient seed order prior to implant, with an average difference of 1.2 ±1.1% from vendor-specified activity. This was documented by a medical physicist and included in the patient’s chart. The un-assayed sources for implant remained in the sterile packaging from the vendor and were transported to the operating room under the supervision of a medical physicist after the completion of the surgical resection. Cesium-131 storage is restricted and based only upon institutional policies and governmental regulations for storing radioactive material; there are no limitations on environmental conditions such as light or temperature.
Intraoperative procedures
Implants were performed intraoperatively, immediately following tumor resection. Patients underwent maximally safe resection of the tumor as determined by the otorhinolaryngology surgeon. At the time of resection, the location and size of the removed tumor (maximum diameter and volume), the relationship to the surrounding normal structures, and the frozen surgical margins were reviewed and compared to the pre-implant treatment plan.
The number of sources was verified for each patient by the AU (authorized user – a qualified radiation oncologist) and medical physicist intraoperatively prior to insertion. The surgical bed was carefully explored by the radiation oncology and surgical oncology teams, and the carotid artery or other major vessels, bones, or cranial nerves were identified. In order to reduce the risk of carotid rupture, osteoradionecrosis, and neuropathies, the seeds were carefully positioned into the surgical bed, avoiding direct contact with the carotid artery, bone, or cranial nerves. The surgeon and radiation oncologist (AU) placed the 131Cs seeds in an appropriate orientation within the resection bed. The seed strands or mesh were secured with surgical clips or sutured by the otorhinolaryngology surgeon and the surgical team continued with the standard wound closure and/or flap reconstruction (Figure 2).
Fig. 2
A) Five strands of either three or four seeds, totalling 18 seeds, were implanted for patient 6 following the neck dissection for locoregional recurrence; B) A pec flap was used in this case for reconstruction to bring radiation- naïve tissue to the resection bed
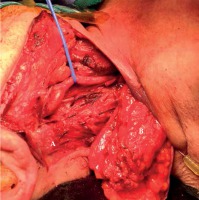
Flap reconstructions are performed in some HNC resection cases regardless of the use of brachytherapy implants; they did not represent additional interventions in these patients. Preparation of the flap reconstruction was performed before the seeds were implanted into the surgical bed in order to reduce the exposure of the surgical team. Flap coverage is the standard of care for head and neck reconstruction for salvage surgery of patients with recurrent disease, having previously received radiation to the surgical site in order to reduce the risk of fistula formation and tissue breakdown post-operatively. Free flaps or pedicled myofascial flaps covered the brachytherapy implants and brought radiation-naïve tissue to the resection bed to promote wound healing. Standard monitoring of the reconstructed flap and/or surgical wound followed the standard institutional protocol.
Although the prescription dose and number of seeds were calculated during a preoperative planning session, the number of seeds implanted was modified intraoperatively to fit the surgical defect and the at-risk area to be covered in some cases. Modifications were determined jointly by the radiation oncology and surgical oncology teams. Additionally, in cases where modifications were large, the radiation prescription dose was also lowered intraoperatively.
Implanted number and activity of seeds were documented on the written directive completed at the end of the implant by the AU and the medical physicist. Sources not used in the procedure were returned to the radiation oncology radioactive storage area, logged in the radiation source inventory, and stored for decay per existing institutional policies.
Post-implant treatment planning and dose calculation
A non-contrast CT scan with 0.7-2.0 mm thick slices and O-MAR artifact reduction protocol was performed within 24 hours after the implant for all patients. Patients 2-5, 10, and 14 were scanned on a Philips Brilliance 64 scanner with 2.0 mm thick slices. Patients 1, 6-9, 11-13, and 15 were scanned on a Philips iCT 256 scanner with 0.7-2.0 mm thick slices. The implanted seeds were identified on the post-implant CT, and a post-implant treatment plan was created to confirm the seed location and delivered prescription dose. Figure 3 displays the pre-implant and post-implant treatment plan for patient 6.
Fig. 3
A) The pre-treatment treatment plan for patient 6. Seed activity of 2.1 U was selected to deliver 60 Gy to 0.5 cm from the center of the implant plane; B) The post-implant treatment plan in created by identifying the seeds on the post-implant CT scan. In this case, the number of implant seeds and the prescription dose did not change based on intraoperative findings
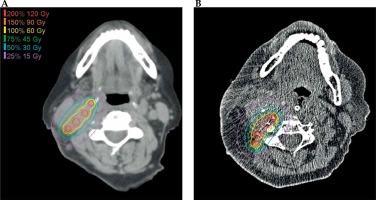
Radiation safety
Cesium-131 was added to our institution’s radiation safety license isotope list for quantities necessary for permanent implant brachytherapy for patients with recurrent resectable HNC. Radiation oncologists were defined as AU by the radiation safety office, in accordance with state legislature and institutional policy for competency in manual brachytherapy. Nursing staff, residents, and physicians participating in clinical care for these patients in the operating room, recovery area, or hospital floor received radiation safety refresher training with an emphasis on the properties of the 131Cs isotope and the implanted sites of the head and neck region. Median equivalent dose to surgeons’ hands was measured using a ring badge for each of the first six cases individually. For subsequent cases, the surgeons were assigned ring badges, which were read quarterly. Additional staff were assigned body or ring dosimeters as deemed appropriate by radiation safety.
Pre- and post-implant radiation surveys of the patient were performed using a Fluke 451B ionization chamber survey meter by the medical physicist. The post-implant survey was performed after final wound closure. Radiation exposure was measured at 1 m from the surgical site.
Educational information, including instructions regarding radiation safety recommendations were provided to the clinical staff in the operating room. Instructions were subsequently handed off to the recovery room and the medical floor nursing teams. Patients returned to the recovery room, then regular surgical floor, and remained inpatients following the standard surgical recovery protocols. Due to the photon energy of 131Cs and implanted activity needed to achieve the desired therapeutic doses, no special room or patient precautions were necessary as all patients met the United States Nuclear Regulatory Commission (NRC) criteria for release of patients administered radioactive material. No shielding was added to the implant site in any of these 15 patients. All patients were educated prior to implantation on the risk and safety concerns regarding radiation exposure. Patients were provided written educational information as part of their discharge instructions regarding radiation safety recommendations.
Statistical methods
The mean and standard deviation were calculated for the number of seeds, individual seed strength, and final prescription dose for 15 patients. A range of minimum-maximum values was also reported. Generally, for radiation safety and personnel dosimetry, mean, standard deviation, and range are reported for the patient survey for 15 implants, and the mean and range of the surgeons’ ring badge reading are reported for the first six cases.
Results
The average seed strength used for implants was 2.2 ±0.2 U (range, 1.7-2.5 U; 3.5 ±0.3 mCi; range, 2.7-3.9 mCi). In five of 15 cases, the number of seeds implanted was modified intraoperatively to fit the surgical defect and the at-risk area to be covered. In three cases, the radiation prescription was lowered intraoperatively. In two of those three cases, the change in number seeds implanted necessitated a prescription change. In the other case (patient 14), it was initially planned that 17 seeds would be implanted in a single site to deliver 60 Gy. However, intraoperative findings led the surgical and radiation oncology teams to alter the prescription such that 40 Gy was delivered to two separate sites, with 6 seeds in one location and 11 in the other. The average final prescription dose for 15 patients was 56.1 ±6.6 Gy (range, 40-60 Gy) to 5 mm from the center of the implanted plane, and an average of 14 ±6 (range, 4-24) seeds were implanted. Table 1 presents the seed strength, and the pre- and post-implant dose prescriptions as well as number of seeds used. Intraoperative changes in early cases were attributed to the learning curve of the clinicians; improved communication between the surgeon and the radiation oncology teams about the estimated size and geometry of the resection cavity decreased the number of deviations between pre- and post-planned prescriptions and the number of seeds implanted.
Table 1
Seed activity, dose prescription, and number of seeds from the pre- and post-plans for all 15 patients, listed in chronological order. Intraoperative changes in the dose prescriptions and number of seeds are indicated
Radiation safety
For the pre-implant survey, the exposure rate at 1 m was < 0.1 mR/hr for all patients, which is the expected background level of radiation. Following implantation of the radioactive sources and conclusion of the surgery, the exposure rate was measured again at 1 m from the surgical site, yielding an average of 1.5 ±1.0 mR/hr (0.1-4.0 mR/hr). Median equivalent dose to surgeons’ hands was 0.60 mSv (range, 0.33-1.48 mSv) for the first six cases. Subsequent quarterly thermoluminescent dosimetry (TLD) ring and optically stimulated luminescence (OSL) body badge measurements were well below acceptable ALARA (as low as reasonably achievable) dose limits.
Discussion
Surgical resection, when feasible, remains the mainstay treatment for recurrent HNC, and intraoperative interstitial brachytherapy delivered as an adjuvant treatment for this group of patients may improve the treatment outcome and may be associated with fewer complications, when compared with EBRT re-irradiation [30,31].
The use of interstitial brachytherapy remains limited in recurrent HNC despite numerous successful published reports. This is perhaps due to the lack of familiarity and experience with implantation techniques and/or concerns about radiation exposure to personnel. Brachytherapy can be delivered via permanent implants or via the insertion of high-activity radioisotopes through removable catheters (using high-dose-rate brachytherapy or pulsed-dose-rate brachytherapy). Permanent implants may be more appropriate and advantageous for recurrent HNC patients, as their recurrences often occur in irregular surfaces that may not be suitable for or may pose challenges for catheter-based brachytherapy [31,32].
Moreover, recent success with safer ultra-low dose-rate isotopes has re-stimulated interest in permanent interstitial brachytherapy and prompted our use in recurrent head and neck malignancies [33,34,35,36].
Cesium-131 is a favorable isotope for use across a wide range of tumors and its dose distribution properties facilitate easy calculation and delivery in the clinic [23,24]. Cesium-131 decays via electron-capture and generates photons with prominent peaks in the 29 to 34 keV range. The average photon energy is greater than other commonly used permanent seed implant isotopes, namely Palladium-103 (103Pd) and Iodine-125 (125I), which has been shown to improve dose homogeneity in some implants [37]. Moreover, 131Cs has a half-life of 9.7 days, which is shorter than 103Pd and 125I, delivering the prescribed dose in less time and decreasing the overall exposure to the patient and family. Additionally, a shorter half-life improves the quality of the implant by delivering dose faster and limiting the time period where seed migration caused by wound healing could interfere with the planed dose distribution [23,24,37].
A major benefit of brachytherapy over external beam radiation therapy in previously irradiated areas is the extremely localized deposition of dose. With 131Cs, the dose is prescribed to 0.5 cm from the implant plane, and the dose falls off to less than 10% of prescription, approximately 2-3 cm in all directions from the implant plane. This dose fall-off and the associated preservation of healthy tissues is unachievable even with the most technologically advanced external beam radiation delivery techniques [38].
While pre-planning, it is necessary to estimate the seed strength and the number of seeds to be implanted; the extent of the surgical resection can result in drastic anatomical changes from the pre-implant imaging. Thus, the number of seeds implanted based on the size and location of the surgical cavity was adjusted intraoperatively in five of these first fifteen cases, and the prescription dose was decreased in three of the five adjusted cases. This reflects the learning curve in anticipating the geometry of the resection cavity and emphasizes the need for communication with the surgical team for the purpose of pre-planning and seed ordering.
The risk of radiation exposure to the health care personnel and patient family members is negligible, and the use of 131Cs brachytherapy does not require additional shielding or patient precautions based on the prescription doses, the total implanted activity, and the location of sources used in these patients. Appropriate educational information is provided to the health care professionals taking care of these patients intra- and post-operatively. Discharge instructions regarding radiation safety recommendations are given to the patients and their family. Our radiation safety data is promising and coincides with the previously published experience. Parashar et al. treated 28 patients with recurrent HNC and brain tumors, using 131Cs sources with a median activity of 2.4 U for a total prescribed dose of 60-80 Gy. In this cohort, the median radiation exposure rate at the patient skin was 0.43 mSv/hr and at 1 meter the exposure was 0.002 mSv/hr. The minimum measurable radiation dose for OSL body badges and TLD ring badges was 1 mrem and 30 mrem, respectively. Overall, the exposure to radiation oncologist, surgeons, and nursing staff was determined to be minimal [24].
Implementation of this procedure requires minimal equipment and staff resources if an existing brachytherapy program is in place. Most low-dose-rate brachytherapy software programs are capable of calculating a basic treatment plan or secondary dose verification for this procedure. Additionally, a nomogram may be an acceptable substitute for a secondary dose calculation in lieu of a formal dose calculation [28]. Point dose dosimetry was applied uniformly to all pre- and post-planning due to the nature of source placement and the inability to accurately decipher source orientation on post-implant imaging. While permanent implant brachytherapy is common in radiation oncology, this may be a new procedure for other clinical staff involved in an HNC patient’s care. Thus, an appropriate introduction into radiation safety and permanent seed implants across the care team is essential.
The radiation dose prescribed by our group concurs with that used by other radiation oncology centers in the setting of resectable recurrent HNC. A group at MD Anderson published their work using 192Ir interstitial brachytherapy with median dose of 60 Gy over a total duration of 4 days, with salvage surgery for 22 patients with recurrent head and neck cancers [33]. All patients underwent neck dissection and intraoperative placement of high-dose-rate brachytherapy afterloading catheters as a part of the salvage treatment. Of the 22 patients, 19 had extensive extracapsular spread at the time of surgery. Post-operative complications were few, and there were no perioperative deaths. Recurrences in the re-irradiated necks occurred in 27% of patients. The 2-year regional control reported by this study was 57%, with a median time for recurrent disease of 19 months [33]. Grimard et al. found similar results by using brachytherapy for recurrent head and neck malignancies; irradiation with brachytherapy was used as a single-modality treatment in almost one half of the patients, with a dose of 60 Gy received by 18 of 22 patients (with all patients receiving > 55 Gy). Twenty of the remaining patients had surgical resection in addition to brachytherapy; the 1 year and 2 years locoregional control was 50% and 37%, respectively, with acceptable rates of toxicity [34]. Puthawala et al. used 192Ir interstitial afterloading brachytherapy delivering a median minimum dose of 53 Gy without surgery (40% received concurrent chemotherapy or a radiosensitizing agent) in the setting of recurrent neck disease, demonstrating a six month local tumor control of 77%; however, moderate to severe complications occurred in 27% of the patients [35]. Similarly, Glatzel et al. reported a complete remission rate of only 28% in 51 patients with recurrent HNC treated with high-dose-rate brachytherapy alone without surgical resection, with a median overall survival of only 6 months [36].
A new technique, intensity modulated brachytherapy (IMBT) has been used with promising results for the treatment of sinonasal carcinomas and tumors invading the skull base and recurrent HNC. The image-guided adapted planning ensures the correct radiation sources position and respects the dosimetric constrains to the surroundings normal structures, possibly further reducing the risk of severe complications [10,13,39]. Teudt et al. used HDR-IMBT for nine patients with recurrent HNC into the cervical lymph nodes [10].
The 131Cs seed implant is permanent and unlike high-dose-rate brachytherapy, does not require the catheters to be placed into the surgical bed and maintained in place for several days post-operatively until treatment is complete. This permanent implant reduces the risk of infection by eliminating the need for in-place catheters and additional surgical intervention to remove them. Surgical resection and the 131Cs implant occur during the same surgical procedure, and no further surgical intervention is required. The surgical bed is defined by the surgical and radiation oncology teams intraoperatively, and the seeds strands are secured into the surgical bed, at that time. The technique is easy to implement and requires minimal equipment and staff resources; the risk of radiation exposure to the health care personnel and patient family members is negligible. From our data and that in previously published literature, the use of 131Cs brachytherapy does not require additional shielding or patient precautions; however, this may depend on the position of sources and the amount of surrounding tissue [23,24]. A disadvantage of permanent implant brachytherapy compared to other forms of brachytherapy or EBRT is the inability to modulate or control the dose distribution.
Re-irradiation using external beam radiation may be used alone or in combination with surgery or systemic therapies, but is associated with significant risk for fistula formation, severe dysphagia, and carotid ruptures, as previously discussed [40,41,42].
Prospective clinical trials eliminating the patient’s tumor and treatment characteristics’ heterogeneity are necessary, but unlikely to be done given the rare use of these options. The final therapeutic choice should depend on a careful review of each individual case in a multidisciplinary treatment approach setting, paying attention to the general performance status of the patient and his/her emotional condition, tumor location and its relationship with the surrounding critical structures, previous radiotherapy dose delivered to this area, the experience of the treating team, and the available treatment facilities.
Conclusions
Cesium-131 interstitial brachytherapy is a feasible treatment option for previously irradiated patients with recurrent, resectable HNC. It should be considered as a viable alternative to external beam radiation. Permanent seed implants using 131Cs do not pose radiation safety concerns to the surgical and nursing staffs, or to patients and their family members. Although our current work focuses on the process of safely treating locoregional recurrent, resectable HNC patients with 131Cs brachytherapy, future work will emphasize the utilization of this form of therapy as a viable treatment option overcoming the negative side effects of re-irradiation using external beam with or without the combination of surgery and systemic therapies for the same patient population.